Molecular modeling, organ culture and reverse genetics for a newly identified human rhinovirus C
- PMID: 21483405
- PMCID: PMC3089712
- DOI: 10.1038/nm.2358
Molecular modeling, organ culture and reverse genetics for a newly identified human rhinovirus C
Abstract
A recently recognized human rhinovirus species C (HRV-C) is associated with up to half of HRV infections in young children. Here we propagated two HRV-C isolates ex vivo in organ culture of nasal epithelial cells, sequenced a new C15 isolate and developed the first, to our knowledge, reverse genetics system for HRV-C. Using contact points for the known HRV receptors, intercellular adhesion molecule-1 (ICAM-1) and low-density lipoprotein receptor (LDLR), inter- and intraspecies footprint analyses predicted a unique cell attachment site for HRV-Cs. Antibodies directed to binding sites for HRV-A and -B failed to inhibit HRV-C attachment, consistent with the alternative receptor footprint. HRV-A and HRV-B infected HeLa and WisL cells but HRV-C did not. However, HRV-C RNA synthesized in vitro and transfected into both cell types resulted in cytopathic effect and recovery of functional virus, indicating that the viral attachment mechanism is a primary distinguishing feature of HRV-C.
Conflict of interest statement
J.E.G. has stock options in EraGen BioSciences (Respiratory Multicode PLx Assay).
Figures
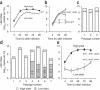
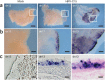
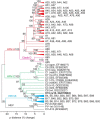
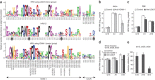
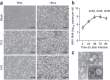
Similar articles
-
Rhinovirus infection of primary cultures of human tracheal epithelium: role of ICAM-1 and IL-1beta.Am J Physiol. 1997 Oct;273(4):L749-59. doi: 10.1152/ajplung.1997.273.4.L749. Am J Physiol. 1997. PMID: 9357849
-
Infection and propagation of human rhinovirus C in human airway epithelial cells.J Virol. 2012 Dec;86(24):13524-32. doi: 10.1128/JVI.02094-12. Epub 2012 Oct 3. J Virol. 2012. PMID: 23035218 Free PMC article.
-
Expression of intercellular adhesion molecule-1 (ICAM-1) in nasal epithelial cells of atopic subjects: a mechanism for increased rhinovirus infection?Clin Exp Immunol. 2000 Aug;121(2):339-45. doi: 10.1046/j.1365-2249.2000.01301.x. Clin Exp Immunol. 2000. PMID: 10931151 Free PMC article.
-
Analysis of the complete genome sequences of human rhinovirus.J Allergy Clin Immunol. 2010 Jun;125(6):1190-9; quiz 1200-1. doi: 10.1016/j.jaci.2010.04.010. Epub 2010 May 14. J Allergy Clin Immunol. 2010. PMID: 20471068 Free PMC article. Review.
-
Uncoating of human rhinoviruses.Rev Med Virol. 2010 Sep;20(5):281-97. doi: 10.1002/rmv.654. Rev Med Virol. 2010. PMID: 20629045 Review.
Cited by
-
Asthma as a chronic disease of the innate and adaptive immune systems responding to viruses and allergens.J Clin Invest. 2012 Aug;122(8):2741-8. doi: 10.1172/JCI60325. Epub 2012 Aug 1. J Clin Invest. 2012. PMID: 22850884 Free PMC article. Review.
-
A molecular epidemiological perspective of rhinovirus types circulating in Amsterdam from 2007 to 2012.Clin Microbiol Infect. 2016 Dec;22(12):1002.e9-1002.e14. doi: 10.1016/j.cmi.2016.08.007. Epub 2016 Aug 21. Clin Microbiol Infect. 2016. PMID: 27554204 Free PMC article.
-
Niclosamide is a proton carrier and targets acidic endosomes with broad antiviral effects.PLoS Pathog. 2012;8(10):e1002976. doi: 10.1371/journal.ppat.1002976. Epub 2012 Oct 25. PLoS Pathog. 2012. PMID: 23133371 Free PMC article.
-
The impact of viral genotype on pathogenesis and disease severity: respiratory syncytial virus and human rhinoviruses.Curr Opin Immunol. 2013 Dec;25(6):761-8. doi: 10.1016/j.coi.2013.09.016. Curr Opin Immunol. 2013. PMID: 24455766 Free PMC article. Review.
-
A pilot study on primary cultures of human respiratory tract epithelial cells to predict patients' responses to H7N9 infection.Oncotarget. 2018 Feb 20;9(18):14492-14508. doi: 10.18632/oncotarget.24537. eCollection 2018 Mar 6. Oncotarget. 2018. PMID: 29581859 Free PMC article.
References
Publication types
MeSH terms
Substances
Grants and funding
LinkOut - more resources
Full Text Sources
Other Literature Sources
Miscellaneous