In vivo positron emission tomographic imaging of glial responses to amyloid-beta and tau pathologies in mouse models of Alzheimer's disease and related disorders
- PMID: 21430171
- PMCID: PMC3251921
- DOI: 10.1523/JNEUROSCI.3076-10.2011
In vivo positron emission tomographic imaging of glial responses to amyloid-beta and tau pathologies in mouse models of Alzheimer's disease and related disorders
Abstract
Core pathologies of Alzheimer's disease (AD) are aggregated amyloid-β peptides (Aβ) and tau, and the latter is also characteristic of diverse neurodegenerative tauopathies. These amyloid lesions provoke microglial activation, and recent neuroimaging technologies have enabled visualization of this response in living brains using radioligands for the peripheral benzodiazepine receptor also known as the 18 kDa translocator protein (TSPO). Here, we elucidated contributions of Aβ and tau deposits to in vivo TSPO signals in pursuit of mechanistic and diagnostic significance of TSPO imaging in AD and other tauopathies. A new antibody to human TSPO revealed induction of TSPO-positive microgliosis by tau fibrils in tauopathy brains. Emergence of TSPO signals before occurrence of brain atrophy and thioflavin-S-positive tau amyloidosis was also demonstrated in living mice transgenic for mutant tau by positron emission tomography (PET) with two classes of TSPO radioligands, [(11)C]AC-5216 and [(18)F]fluoroethoxy-DAA1106. Meanwhile, only modest TSPO elevation was observed in aged mice modeling Aβ plaque deposition, despite the notably enhanced in vivo binding of amyloid radiotracer, [(11)C]Pittsburgh Compound-B, to plaques. In these animals, [(11)C]AC-5216 yielded better TSPO contrasts than [(18)F]fluoroethoxy-DAA1106, supporting the possibility of capturing early neurotoxicity with high-performance TSPO probes. Furthermore, an additional line of mice modeling intraneuronal Aβ accumulation displayed elevated TSPO signals following noticeable neuronal loss, unlike TSPO upregulation heralding massive neuronal death in tauopathy model mice. Our data corroborate the utility of TSPO-PET imaging as a biomarker for tau-triggered toxicity, and as a complement to amyloid scans for diagnostic assessment of tauopathies with and without Aβ pathologies.
Figures
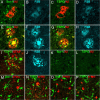
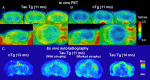
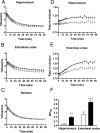
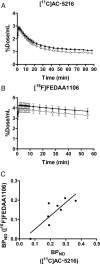
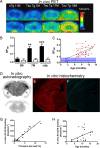
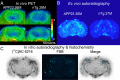
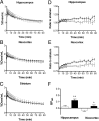
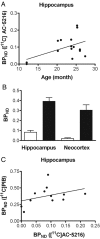
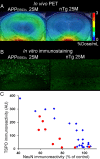
Similar articles
-
Neuroprotective effect of mitochondrial translocator protein ligand in a mouse model of tauopathy.J Neuroinflammation. 2021 Mar 19;18(1):76. doi: 10.1186/s12974-021-02122-1. J Neuroinflammation. 2021. PMID: 33740987 Free PMC article.
-
Distinct binding of amyloid imaging ligands to unique amyloid-β deposited in the presubiculum of Alzheimer's disease.J Neurochem. 2015 Dec;135(5):859-66. doi: 10.1111/jnc.13293. Epub 2015 Sep 17. J Neurochem. 2015. PMID: 26315807
-
Visualization of brain amyloid and microglial activation in mouse models of Alzheimer's disease.Curr Alzheimer Res. 2009 Apr;6(2):137-43. doi: 10.2174/156720509787602906. Curr Alzheimer Res. 2009. PMID: 19355848
-
Molecular imaging of dementia.Psychogeriatrics. 2012 Jun;12(2):106-14. doi: 10.1111/j.1479-8301.2012.00409.x. Psychogeriatrics. 2012. PMID: 22712644 Review.
-
[Development of SPECT Probes for In Vivo Imaging of β-Amyloid and Tau Aggregates in the Alzheimer's Disease Brain].Yakugaku Zasshi. 2017;137(11):1361-1365. doi: 10.1248/yakushi.17-00156. Yakugaku Zasshi. 2017. PMID: 29093372 Review. Japanese.
Cited by
-
Neuroprotective effect of mitochondrial translocator protein ligand in a mouse model of tauopathy.J Neuroinflammation. 2021 Mar 19;18(1):76. doi: 10.1186/s12974-021-02122-1. J Neuroinflammation. 2021. PMID: 33740987 Free PMC article.
-
Imaging of tau pathology in a tauopathy mouse model and in Alzheimer patients compared to normal controls.Neuron. 2013 Sep 18;79(6):1094-108. doi: 10.1016/j.neuron.2013.07.037. Neuron. 2013. PMID: 24050400 Free PMC article.
-
Huperzine A: Is it an Effective Disease-Modifying Drug for Alzheimer's Disease?Front Aging Neurosci. 2014 Aug 19;6:216. doi: 10.3389/fnagi.2014.00216. eCollection 2014. Front Aging Neurosci. 2014. PMID: 25191267 Free PMC article. Review.
-
Microglial Activity in People at Ultra High Risk of Psychosis and in Schizophrenia: An [(11)C]PBR28 PET Brain Imaging Study.Am J Psychiatry. 2016 Jan;173(1):44-52. doi: 10.1176/appi.ajp.2015.14101358. Epub 2015 Oct 16. Am J Psychiatry. 2016. PMID: 26472628 Free PMC article.
-
PET imaging of inflammation biomarkers.Theranostics. 2013 Jun 24;3(7):448-66. doi: 10.7150/thno.6592. Print 2013. Theranostics. 2013. PMID: 23843893 Free PMC article. Review.
References
-
- Block ML, Zecca L, Hong JS. Microglia-mediated neurotoxicity: uncovering the molecular mechanisms. Nat Rev Neurosci. 2007;8:57–69. - PubMed
-
- Cagnin A, Brooks DJ, Kennedy AM, Gunn RN, Myers R, Turkheimer FE, Jones T, Banati RB. In-vivo measurement of activated microglia in dementia. Lancet. 2001;358:461–467. - PubMed
-
- Diorio D, Welner SA, Butterworth RF, Meaney MJ, Suranyi-Cadotte BE. Peripheral benzodiazepine binding sites in Alzheimer's disease frontal and temporal cortex. Neurobiol Aging. 1991;12:255–258. - PubMed
Publication types
MeSH terms
Substances
Grants and funding
LinkOut - more resources
Full Text Sources
Other Literature Sources
Medical
Molecular Biology Databases
Miscellaneous