Scraping through the ice: uncovering the role of TRPM8 in cold transduction
- PMID: 21411765
- PMCID: PMC3119154
- DOI: 10.1152/ajpregu.00631.2010
Scraping through the ice: uncovering the role of TRPM8 in cold transduction
Abstract
The proper detection of environmental temperatures is essential for the optimal growth and survival of organisms of all shapes and phyla, yet only recently have the molecular mechanisms for temperature sensing been elucidated. The discovery of temperature-sensitive ion channels of the transient receptor potential (TRP) superfamily has been pivotal in explaining how temperatures are sensed in vivo, and here we will focus on the lone member of this cohort, TRPM8, which has been unequivocally shown to be cold sensitive. TRPM8 is expressed in somatosensory neurons that innervate peripheral tissues such as the skin and oral cavity, and recent genetic evidence has shown it to be the principal transducer of cool and cold stimuli. It is remarkable that this one channel, unlike other thermosensitive TRP channels, is associated with both innocuous and noxious temperature transduction, as well as cold hypersensitivity during injury and, paradoxically, cold-mediated analgesia. With ongoing research, the field is getting closer to answering a number of fundamental questions regarding this channel, including the cellular mechanisms of TRPM8 modulation, the molecular context of TRPM8 expression, as well as the full extent of the role of TRPM8 in cold signaling in vivo. These findings will further our understanding of basic thermotransduction and sensory coding, and may have important implications for treatments for acute and chronic pain.
Figures
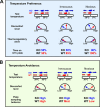
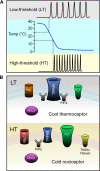
Similar articles
-
Diversity in the neural circuitry of cold sensing revealed by genetic axonal labeling of transient receptor potential melastatin 8 neurons.J Neurosci. 2007 Dec 19;27(51):14147-57. doi: 10.1523/JNEUROSCI.4578-07.2007. J Neurosci. 2007. PMID: 18094254 Free PMC article.
-
A sensory-labeled line for cold: TRPM8-expressing sensory neurons define the cellular basis for cold, cold pain, and cooling-mediated analgesia.J Neurosci. 2013 Feb 13;33(7):2837-48. doi: 10.1523/JNEUROSCI.1943-12.2013. J Neurosci. 2013. PMID: 23407943 Free PMC article.
-
TRPM8, a sensor for mild cooling in mammalian sensory nerve endings.Curr Pharm Biotechnol. 2011 Jan 1;12(1):78-88. doi: 10.2174/138920111793937835. Curr Pharm Biotechnol. 2011. PMID: 20932256 Review.
-
TRPA1 expression levels and excitability brake by KV channels influence cold sensitivity of TRPA1-expressing neurons.Neuroscience. 2017 Jun 14;353:76-86. doi: 10.1016/j.neuroscience.2017.04.001. Epub 2017 Apr 10. Neuroscience. 2017. PMID: 28408328 Free PMC article.
-
Intimacies and physiological role of the polymodal cold-sensitive ion channel TRPM8.Curr Top Membr. 2014;74:293-324. doi: 10.1016/B978-0-12-800181-3.00011-7. Curr Top Membr. 2014. PMID: 25366241 Review.
Cited by
-
Molecular basis of peripheral innocuous cold sensitivity.Handb Clin Neurol. 2018;156:57-67. doi: 10.1016/B978-0-444-63912-7.00003-5. Handb Clin Neurol. 2018. PMID: 30454609 Free PMC article. Review.
-
The molecular and cellular basis of cold sensation.ACS Chem Neurosci. 2013 Feb 20;4(2):238-47. doi: 10.1021/cn300193h. Epub 2012 Nov 28. ACS Chem Neurosci. 2013. PMID: 23421674 Free PMC article. Review.
-
Chemogenetic management of neuropathic pain.Brain. 2017 Oct 1;140(10):2522-2525. doi: 10.1093/brain/awx227. Brain. 2017. PMID: 28969393 Free PMC article.
-
The role of trigeminal nasal TRPM8-expressing afferent neurons in the antitussive effects of menthol.J Appl Physiol (1985). 2013 Jul 15;115(2):268-74. doi: 10.1152/japplphysiol.01144.2012. Epub 2013 May 2. J Appl Physiol (1985). 2013. PMID: 23640596 Free PMC article.
-
Mediators of Chronic Pruritus in Atopic Dermatitis: Getting the Itch Out?Clin Rev Allergy Immunol. 2016 Dec;51(3):263-292. doi: 10.1007/s12016-015-8488-5. Clin Rev Allergy Immunol. 2016. PMID: 25931325 Review.
References
-
- Abrahamsen B, Zhao J, Asante CO, Cendan CM, Marsh S, Martinez-Barbera JP, Nassar MA, Dickenson AH, Wood JN. The cell and molecular basis of mechanical, cold, and inflammatory pain. Science 321: 702–705, 2008 - PubMed
-
- Attal N, Bouhassira D, Gautron M, Vaillant JN, Mitry E, Lepere C, Rougier P, Guirimand F. Thermal hyperalgesia as a marker of oxaliplatin neurotoxicity: a prospective quantified sensory assessment study. Pain 144: 245–252, 2009 - PubMed
Publication types
MeSH terms
Substances
Grants and funding
LinkOut - more resources
Full Text Sources