Conditional ablation of Pten in osteoprogenitors stimulates FGF signaling
- PMID: 21385768
- PMCID: PMC3050668
- DOI: 10.1242/dev.058016
Conditional ablation of Pten in osteoprogenitors stimulates FGF signaling
Abstract
Phosphatase and tensin homolog deleted on chromosome ten (PTEN) is a direct antagonist of phosphatidylinositol 3 kinase. Pten is a well recognized tumor suppressor and is one of the most commonly mutated genes in human malignancies. More recent studies of development and stem cell behavior have shown that PTEN regulates the growth and differentiation of progenitor cells. Significantly, PTEN is found in osteoprogenitor cells that give rise to bone-forming osteoblasts; however, the role of PTEN in bone development is incompletely understood. To define how PTEN functions in osteoprogenitors during bone development, we conditionally deleted Pten in mice using the cre-deleter strain Dermo1cre, which targets undifferentiated mesenchyme destined to form bone. Deletion of Pten in osteoprogenitor cells led to increased numbers of osteoblasts and expanded bone matrix. Significantly, osteoblast development and synthesis of osteoid in the nascent bone collar was uncoupled from the usual tight linkage to chondrocyte differentiation in the epiphyseal growth plate. The expansion of osteoblasts and osteoprogenitors was found to be due to augmented FGF signaling as evidenced by (1) increased expression of FGF18, a potent osteoblast mitogen, and (2) decreased expression of SPRY2, a repressor of FGF signaling. The differentiation of osteoblasts was autonomous from the growth plate chondrocytes and was correlated with an increase in the protein levels of GLI2, a transcription factor that is a major mediator of hedgehog signaling. We provide evidence that increased GLI2 activity is also a consequence of increased FGF signaling through downstream events requiring mitogen-activated protein kinases. To test whether FGF signaling is required for the effects of Pten deletion, we deleted one allele of fibroblast growth factor receptor 2 (FGFR2). Significantly, deletion of FGFR2 caused a partial rescue of the Pten-null phenotype. This study identifies activated FGF signaling as the major mediator of Pten deletion in osteoprogenitors.
Figures
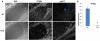
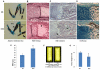
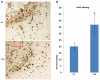
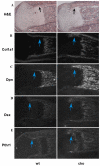
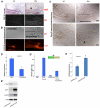
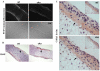
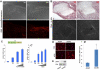
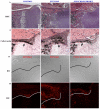
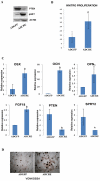
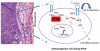
Similar articles
-
Conditional inactivation of FGF receptor 2 reveals an essential role for FGF signaling in the regulation of osteoblast function and bone growth.Development. 2003 Jul;130(13):3063-74. doi: 10.1242/dev.00491. Development. 2003. PMID: 12756187
-
FGF signaling in the osteoprogenitor lineage non-autonomously regulates postnatal chondrocyte proliferation and skeletal growth.Development. 2016 May 15;143(10):1811-22. doi: 10.1242/dev.131722. Epub 2016 Apr 6. Development. 2016. PMID: 27052727 Free PMC article.
-
Regulation of osteoblast, chondrocyte, and osteoclast functions by fibroblast growth factor (FGF)-18 in comparison with FGF-2 and FGF-10.J Biol Chem. 2002 Mar 1;277(9):7493-500. doi: 10.1074/jbc.M108653200. Epub 2001 Dec 11. J Biol Chem. 2002. PMID: 11741978
-
Fibroblast growth factor signaling controlling osteoblast differentiation.Gene. 2003 Oct 16;316:23-32. doi: 10.1016/s0378-1119(03)00748-0. Gene. 2003. PMID: 14563548 Review.
-
Regulation of bone development and extracellular matrix protein genes by RUNX2.Cell Tissue Res. 2010 Jan;339(1):189-95. doi: 10.1007/s00441-009-0832-8. Epub 2009 Aug 1. Cell Tissue Res. 2010. PMID: 19649655 Review.
Cited by
-
Fibulin-1 is required for bone formation and Bmp-2-mediated induction of Osterix.Bone. 2014 Dec;69:30-8. doi: 10.1016/j.bone.2014.07.038. Epub 2014 Sep 6. Bone. 2014. PMID: 25201465 Free PMC article.
-
Lens fiber cell differentiation occurs independently of fibroblast growth factor receptor signaling in the absence of Pten.Dev Biol. 2020 Nov 1;467(1-2):1-13. doi: 10.1016/j.ydbio.2020.07.017. Epub 2020 Aug 25. Dev Biol. 2020. PMID: 32858001 Free PMC article.
-
Loss of Nmp4 optimizes osteogenic metabolism and secretion to enhance bone quality.Am J Physiol Endocrinol Metab. 2019 May 1;316(5):E749-E772. doi: 10.1152/ajpendo.00343.2018. Epub 2019 Jan 15. Am J Physiol Endocrinol Metab. 2019. PMID: 30645175 Free PMC article.
-
A specific role for phosphoinositide 3-kinase and AKT in osteoblasts?Front Endocrinol (Lausanne). 2012 Jul 20;3:88. doi: 10.3389/fendo.2012.00088. eCollection 2012. Front Endocrinol (Lausanne). 2012. PMID: 22833734 Free PMC article.
-
FGFR and PTEN signaling interact during lens development to regulate cell survival.Dev Biol. 2016 Feb 15;410(2):150-163. doi: 10.1016/j.ydbio.2015.12.027. Epub 2016 Jan 5. Dev Biol. 2016. PMID: 26764128 Free PMC article.
References
-
- Brewster R., Mullor J. L., Ruiz i Altaba A. (2000). Gli2 functions in FGF signaling during antero-posterior patterning. Development 127, 4395-4405 - PubMed
-
- Brunet A., Bonni A., Zigmond M. J., Lin M. Z., Juo P., Hu L. S., Anderson M. J., Arden K. C., Blenis J., Greenberg M. E. (1999). Akt promotes cell survival by phosphorylating and inhibiting a Forkhead transcription factor. Cell 96, 857-868 - PubMed
-
- Colnot C., Lu C., Hu D., Helms J. A. (2004). Distinguishing the contributions of the perichondrium, cartilage, and vascular endothelium to skeletal development. Dev. Biol. 269, 55-69 - PubMed
-
- Cooper M. K., Wassif C. A., Krakowiak P. A., Taipale J., Gong R., Kelley R. I., Porter F. D., Beachy P. A. (2003). A defective response to Hedgehog signaling in disorders of cholesterol biosynthesis. Nat. Genet. 33, 508-513 - PubMed
Publication types
MeSH terms
Substances
Grants and funding
LinkOut - more resources
Full Text Sources
Other Literature Sources
Molecular Biology Databases
Research Materials
Miscellaneous