Large-scale calcium waves traveling through astrocytic networks in vivo
- PMID: 21325528
- PMCID: PMC6623677
- DOI: 10.1523/JNEUROSCI.5319-10.2011
Large-scale calcium waves traveling through astrocytic networks in vivo
Abstract
Macroscopic changes in cerebral blood flow, such as those captured by functional imaging of the brain, require highly organized, large-scale dynamics of astrocytes, glial cells that interact with both neuronal and cerebrovascular networks. However, astrocyte activity has been studied mainly at the level of individual cells, and information regarding their collective behavior is lacking. In this work, we monitored calcium activity simultaneously from hundreds of mouse hippocampal astrocytes in vivo and found that almost all astrocytes participated en masse in regenerative waves that propagated from cell to cell (referred to here as "glissandi"). Glissandi emerged depending on the neuronal activity and accompanied a reduction in infraslow fluctuations of local field potentials and a decrease in the flow of red blood cells. This novel phenomenon was heretofore overlooked, probably because of the high vulnerability of astrocytes to light damage; glissandi occurred only when observed at much lower laser intensities than previously used.
Figures
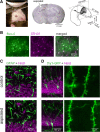
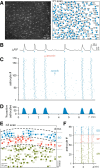
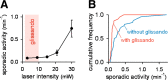
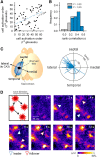
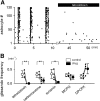
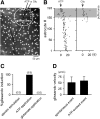
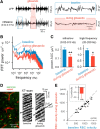
Similar articles
-
Properties of synaptically evoked astrocyte calcium signal reveal synaptic information processing by astrocytes.J Neurosci. 2005 Mar 2;25(9):2192-203. doi: 10.1523/JNEUROSCI.3965-04.2005. J Neurosci. 2005. PMID: 15745945 Free PMC article.
-
Astrocytes display complex and localized calcium responses to single-neuron stimulation in the hippocampus.J Neurosci. 2011 Jun 15;31(24):8905-19. doi: 10.1523/JNEUROSCI.6341-10.2011. J Neurosci. 2011. PMID: 21677174 Free PMC article.
-
Astrocytes in 17beta-estradiol treated mixed hippocampal cultures show attenuated calcium response to neuronal activity.Glia. 2006 Jun;53(8):817-26. doi: 10.1002/glia.20341. Glia. 2006. PMID: 16565986
-
Bidirectional astrocyte-neuron communication: the many roles of glutamate and ATP.Novartis Found Symp. 2006;276:208-17; discussion 217-21, 233-7, 275-81. doi: 10.1002/9780470032244.ch16. Novartis Found Symp. 2006. PMID: 16805432 Review.
-
The cellular basis of neurovascular metabolic coupling.Acta Neurol Belg. 2002 Dec;102(4):153-7. Acta Neurol Belg. 2002. PMID: 12534240 Review.
Cited by
-
Axon growth and synaptic function: A balancing act for axonal regeneration and neuronal circuit formation in CNS trauma and disease.Dev Neurobiol. 2020 Jul;80(7-8):277-301. doi: 10.1002/dneu.22780. Epub 2020 Sep 28. Dev Neurobiol. 2020. PMID: 32902152 Free PMC article. Review.
-
The computational power of astrocyte mediated synaptic plasticity.Front Comput Neurosci. 2012 Nov 1;6:93. doi: 10.3389/fncom.2012.00093. eCollection 2012. Front Comput Neurosci. 2012. PMID: 23125832 Free PMC article.
-
Sedentary Life and Reduced Mastication Impair Spatial Learning and Memory and Differentially Affect Dentate Gyrus Astrocyte Subtypes in the Aged Mice.Front Neurosci. 2021 Apr 15;15:632216. doi: 10.3389/fnins.2021.632216. eCollection 2021. Front Neurosci. 2021. PMID: 33935629 Free PMC article.
-
Infraslow coordination of slow wave activity through altered neuronal synchrony.Sleep. 2019 Dec 24;42(12):zsz170. doi: 10.1093/sleep/zsz170. Sleep. 2019. PMID: 31353415 Free PMC article.
-
Impact of DC-Coupled Electrophysiological Recordings for Translational Neuroscience: Case Study of Tracking Neural Dynamics in Rodent Models of Seizures.Front Comput Neurosci. 2022 Jul 21;16:900063. doi: 10.3389/fncom.2022.900063. eCollection 2022. Front Comput Neurosci. 2022. PMID: 35936824 Free PMC article.
References
-
- Agulhon C, Fiacco TA, McCarthy KD. Hippocampal short- and long-term plasticity are not modulated by astrocyte Ca2+ signaling. Science. 2010;327:1250–1254. - PubMed
Publication types
MeSH terms
Substances
LinkOut - more resources
Full Text Sources
Other Literature Sources
Research Materials