Tracking Glideosome-associated protein 50 reveals the development and organization of the inner membrane complex of Plasmodium falciparum
- PMID: 21239623
- PMCID: PMC3127638
- DOI: 10.1128/EC.00244-10
Tracking Glideosome-associated protein 50 reveals the development and organization of the inner membrane complex of Plasmodium falciparum
Abstract
The most deadly of the human malaria parasites, Plasmodium falciparum, has different stages specialized for invasion of hepatocytes, erythrocytes, and the mosquito gut wall. In each case, host cell invasion is powered by an actin-myosin motor complex that is linked to an inner membrane complex (IMC) via a membrane anchor called the glideosome-associated protein 50 (PfGAP50). We generated P. falciparum transfectants expressing green fluorescent protein (GFP) chimeras of PfGAP50 (PfGAP50-GFP). Using immunoprecipitation and fluorescence photobleaching, we show that C-terminally tagged PfGAP50-GFP can form a complex with endogenous copies of the linker protein PfGAP45 and the myosin A tail domain-interacting protein (MTIP). Full-length PfGAP50-GFP is located in the endoplasmic reticulum in early-stage parasites and then redistributes to apical caps during the formation of daughter merozoites. In the final stage of schizogony, the PfGAP50-GFP profile extends further around the merozoite surface. Three-dimensional (3D) structured illumination microscopy reveals the early-stage IMC as a doubly punctured flat ellipsoid that separates to form claw-shaped apposed structures. A GFP fusion of PfGAP50 lacking the C-terminal membrane anchor is misdirected to the parasitophorous vacuole. Replacement of the acid phosphatase homology domain of PfGAP50 with GFP appears to allow correct trafficking of the chimera but confers a growth disadvantage.
Figures
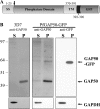
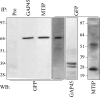
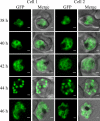
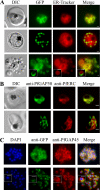
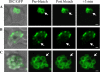
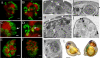
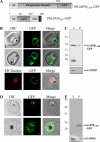
Similar articles
-
Plasmodium falciparum GAP40 Plays an Essential Role in Merozoite Invasion and Gametocytogenesis.Microbiol Spectr. 2023 Jun 15;11(3):e0143423. doi: 10.1128/spectrum.01434-23. Epub 2023 May 30. Microbiol Spectr. 2023. PMID: 37249423 Free PMC article.
-
Effects of calcium signaling on Plasmodium falciparum erythrocyte invasion and post-translational modification of gliding-associated protein 45 (PfGAP45).Mol Biochem Parasitol. 2009 Nov;168(1):55-62. doi: 10.1016/j.molbiopara.2009.06.007. Epub 2009 Jul 1. Mol Biochem Parasitol. 2009. PMID: 19576251 Free PMC article.
-
Plasmodium falciparum erythrocyte invasion: a conserved myosin associated complex.Mol Biochem Parasitol. 2006 May;147(1):74-84. doi: 10.1016/j.molbiopara.2006.01.009. Epub 2006 Feb 13. Mol Biochem Parasitol. 2006. PMID: 16513191
-
Erythrocyte invasion receptors for Plasmodium falciparum: new and old.Transfus Med. 2016 Apr;26(2):77-88. doi: 10.1111/tme.12280. Epub 2016 Feb 9. Transfus Med. 2016. PMID: 26862042 Review.
-
Vesicle-mediated trafficking of parasite proteins to the host cell cytosol and erythrocyte surface membrane in Plasmodium falciparum infected erythrocytes.Int J Parasitol. 2001 Oct;31(12):1381-91. doi: 10.1016/s0020-7519(01)00256-9. Int J Parasitol. 2001. PMID: 11566305 Review.
Cited by
-
Subcellular location, phosphorylation and assembly into the motor complex of GAP45 during Plasmodium falciparum schizont development.PLoS One. 2012;7(3):e33845. doi: 10.1371/journal.pone.0033845. Epub 2012 Mar 30. PLoS One. 2012. PMID: 22479457 Free PMC article.
-
Spatiotemporal and functional characterisation of the Plasmodium falciparum cGMP-dependent protein kinase.PLoS One. 2012;7(11):e48206. doi: 10.1371/journal.pone.0048206. Epub 2012 Nov 5. PLoS One. 2012. PMID: 23139764 Free PMC article.
-
Malaria.tools-comparative genomic and transcriptomic database for Plasmodium species.Nucleic Acids Res. 2020 Jan 8;48(D1):D768-D775. doi: 10.1093/nar/gkz662. Nucleic Acids Res. 2020. PMID: 31372645 Free PMC article.
-
Unique apicomplexan IMC sub-compartment proteins are early markers for apical polarity in the malaria parasite.Biol Open. 2013 Sep 16;2(11):1160-70. doi: 10.1242/bio.20136163. eCollection 2013. Biol Open. 2013. PMID: 24244852 Free PMC article.
-
Disrupting assembly of the inner membrane complex blocks Plasmodium falciparum sexual stage development.PLoS Pathog. 2017 Oct 6;13(10):e1006659. doi: 10.1371/journal.ppat.1006659. eCollection 2017 Oct. PLoS Pathog. 2017. PMID: 28985225 Free PMC article.
References
-
- Abu Bakar N. A., Klonis N., Hanssen E., Chan C., Tilley L. 2010. Digestive-vacuole genesis and endocytic processes in the early intraerythrocytic stages of Plasmodium falciparum. J. Cell Sci. 123:441–450 - PubMed
-
- Adisa A., et al. 2007. Re-assessing the locations of components of the classical vesicle-mediated trafficking machinery in transfected Plasmodium falciparum. Int. J. Parasitol. 37:1127–1141 - PubMed
-
- Adisa A., et al. 2003. The signal sequence of exported protein-1 directs the green fluorescent protein to the parasitophorous vacuole of transfected malaria parasites. J. Biol. Chem. 278:6532–6542 - PubMed
-
- Bannister L. H., Dluzewski A. R. 1990. The ultrastructure of red cell invasion in malaria infections: a review. Blood Cells 16:257–292 - PubMed
-
- Bannister L. H., Hopkins J. M., Fowler R. E., Krishna S., Mitchell G. H. 2000. Ultrastructure of rhoptry development in Plasmodium falciparum erythrocytic schizonts. Parasitology 121:273–287 - PubMed
Publication types
MeSH terms
Substances
LinkOut - more resources
Full Text Sources
Miscellaneous