Multivesicular bodies in neurons: distribution, protein content, and trafficking functions
- PMID: 21216273
- PMCID: PMC3055956
- DOI: 10.1016/j.pneurobio.2011.01.003
Multivesicular bodies in neurons: distribution, protein content, and trafficking functions
Abstract
Multivesicular bodies (MVBs) are intracellular endosomal organelles characterized by multiple internal vesicles that are enclosed within a single outer membrane. MVBs were initially regarded as purely prelysosomal structures along the degradative endosomal pathway of internalized proteins. MVBs are now known to be involved in numerous endocytic and trafficking functions, including protein sorting, recycling, transport, storage, and release. This review of neuronal MVBs summarizes their research history, morphology, distribution, accumulation of cargo and constitutive proteins, transport, and theories of functions of MVBs in neurons and glia. Due to their complex morphologies, neurons have expanded trafficking and signaling needs, beyond those of "geometrically simpler" cells, but it is not known whether neuronal MVBs perform additional transport and signaling functions. This review examines the concept of compartment-specific MVB functions in endosomal protein trafficking and signaling within synapses, axons, dendrites and cell bodies. We critically evaluate reports of the accumulation of neuronal MVBs based on evidence of stress-induced MVB formation. Furthermore, we discuss potential functions of neuronal and glial MVBs in development, in dystrophic neuritic syndromes, injury, disease, and aging. MVBs may play a role in Alzheimer's, Huntington's, and Niemann-Pick diseases, some types of frontotemporal dementia, prion and virus trafficking, as well as in adaptive responses of neurons to trauma and toxin or drug exposure. Functions of MVBs in neurons have been much neglected, and major gaps in knowledge currently exist. Developing truly MVB-specific markers would help to elucidate the roles of neuronal MVBs in intra- and intercellular signaling of normal and diseased neurons.
Copyright © 2011 Elsevier Ltd. All rights reserved.
Figures
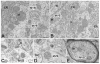
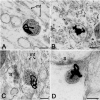
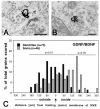
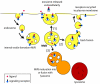
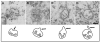
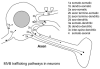
Similar articles
-
Focus on the morphogenesis, fate and the role in tumor progression of multivesicular bodies.Cell Commun Signal. 2020 Aug 8;18(1):122. doi: 10.1186/s12964-020-00619-5. Cell Commun Signal. 2020. PMID: 32771015 Free PMC article. Review.
-
Quantitative analysis of multivesicular bodies (MVBs) in the hypoglossal nerve: evidence that neurotrophic factors do not use MVBs for retrograde axonal transport.J Comp Neurol. 2009 Jun 20;514(6):641-57. doi: 10.1002/cne.22047. J Comp Neurol. 2009. PMID: 19363811 Free PMC article.
-
Multivesicular body morphogenesis.Annu Rev Cell Dev Biol. 2012;28:337-62. doi: 10.1146/annurev-cellbio-092910-154152. Epub 2012 Jul 20. Annu Rev Cell Dev Biol. 2012. PMID: 22831642 Review.
-
Biogenesis of Plant Prevacuolar Multivesicular Bodies.Mol Plant. 2016 Jun 6;9(6):774-86. doi: 10.1016/j.molp.2016.01.011. Epub 2016 Feb 2. Mol Plant. 2016. PMID: 26836198 Review.
-
Size, Shape, and Distribution of Multivesicular Bodies in the Juvenile Rat Somatosensory Cortex: A 3D Electron Microscopy Study.Cereb Cortex. 2020 Mar 14;30(3):1887-1901. doi: 10.1093/cercor/bhz211. Cereb Cortex. 2020. PMID: 31665237 Free PMC article.
Cited by
-
Endocytic control of growth factor signalling: multivesicular bodies as signalling organelles.Nat Rev Mol Cell Biol. 2011 Nov 23;13(1):53-60. doi: 10.1038/nrm3244. Nat Rev Mol Cell Biol. 2011. PMID: 22108513 Free PMC article. Review.
-
Extracellular vesicles: communication, coercion, and conditioning.Mol Biol Cell. 2013 May;24(9):1253-9. doi: 10.1091/mbc.E12-08-0572. Mol Biol Cell. 2013. PMID: 23630232 Free PMC article.
-
Retrograde Transport by Clathrin-Coated Vesicles is Involved in Intracellular Transport of PrPSc in Persistently Prion-Infected Cells.Sci Rep. 2018 Aug 16;8(1):12241. doi: 10.1038/s41598-018-30775-1. Sci Rep. 2018. PMID: 30115966 Free PMC article.
-
ATG9 resides on a unique population of small vesicles in presynaptic nerve terminals.Autophagy. 2024 Apr;20(4):883-901. doi: 10.1080/15548627.2023.2274204. Epub 2023 Nov 8. Autophagy. 2024. PMID: 37881948 Free PMC article.
-
Signaling pathways in exosomes biogenesis, secretion and fate.Genes (Basel). 2013 Mar 28;4(2):152-70. doi: 10.3390/genes4020152. Genes (Basel). 2013. PMID: 24705158 Free PMC article.
References
-
- Agromayor M, Martin-Serrano J. Interaction of AMSH with ESCRT-III and deubiquitination of endosomal cargo. J Biol Chem. 2006;281:23083–23091. - PubMed
-
- Aguado F, Sanchez-Franco F, Cacidedo L, Fernandez T, Rodrigo J, Martinez-Murillo R. Subcellular localization of insulin-like growth factor I (IGF-I) in Purkinje cells of the adult rat: an immunocytochemical study. Neurosci Lett. 1992;135:171–174. - PubMed
-
- Agulhon C, Rostaing P, Ravassard P, Sagne C, Triller A, Giros B. Lysosomal amino acid transporter LYAAT-1 in the rat central nervous system: an in situ hybridization and immunohistochemical study. J Comp Neurol. 2003;462:71–89. - PubMed
-
- Alberts P, Galli T. The cell outgrowth secretory endosome (COSE): a specialized compartment involved in neuronal morphogenesis. Biol Cell. 2003;95:419–424. - PubMed
Publication types
MeSH terms
Substances
Grants and funding
- P20 RR016464/RR/NCRR NIH HHS/United States
- R03 TW005700-03/TW/FIC NIH HHS/United States
- P20RR016464/RR/NCRR NIH HHS/United States
- R01 NS035931-02S1/NS/NINDS NIH HHS/United States
- EY 12841/EY/NEI NIH HHS/United States
- HD 043347/HD/NICHD NIH HHS/United States
- P20 RR016464-09/RR/NCRR NIH HHS/United States
- R01 EY012841/EY/NEI NIH HHS/United States
- R21 NS073113/NS/NINDS NIH HHS/United States
- R03 HD043347/HD/NICHD NIH HHS/United States
- R01 EY012841-10/EY/NEI NIH HHS/United States
- R21 NS073113-01A1/NS/NINDS NIH HHS/United States
LinkOut - more resources
Full Text Sources