Asymmetric ATP hydrolysis cycle of the heterodimeric multidrug ABC transport complex TmrAB from Thermus thermophilus
- PMID: 21190941
- PMCID: PMC3044967
- DOI: 10.1074/jbc.M110.201178
Asymmetric ATP hydrolysis cycle of the heterodimeric multidrug ABC transport complex TmrAB from Thermus thermophilus
Abstract
ATP-binding cassette (ABC) systems translocate a wide range of solutes across cellular membranes. The thermophilic gram-negative eubacterium Thermus thermophilus, a model organism for structural genomics and systems biology, discloses ∼46 ABC proteins, which are largely uncharacterized. Here, we functionally analyzed the first two and only ABC half-transporters of the hyperthermophilic bacterium, TmrA and TmrB. The ABC system mediates uptake of the drug Hoechst 33342 in inside-out oriented vesicles that is inhibited by verapamil. TmrA and TmrB form a stable heterodimeric complex hydrolyzing ATP with a K(m) of 0.9 mm and k(cat) of 9 s(-1) at 68 °C. Two nucleotides can be trapped in the heterodimeric ABC complex either by vanadate or by mutation inhibiting ATP hydrolysis. Nucleotide trapping requires permissive temperatures, at which a conformational ATP switch is possible. We further demonstrate that the canonic glutamate 523 of TmrA is essential for rapid conversion of the ATP/ATP-bound complex into its ADP/ATP state, whereas the corresponding aspartate in TmrB (Asp-500) has only a regulatory role. Notably, exchange of this single noncanonic residue into a catalytic glutamate cannot rescue the function of the E523Q/D500E complex, implicating a built-in asymmetry of the complex. However, slow ATP hydrolysis in the newly generated canonic site (D500E) strictly depends on the formation of a posthydrolysis state in the consensus site, indicating an allosteric coupling of both active sites.
Figures
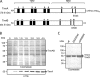
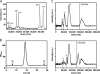
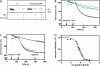
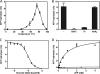
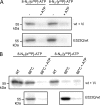
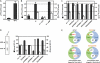
Similar articles
-
A Conserved Motif in Intracellular Loop 1 Stabilizes the Outward-Facing Conformation of TmrAB.J Mol Biol. 2021 Aug 6;433(16):166834. doi: 10.1016/j.jmb.2021.166834. Epub 2021 Jan 29. J Mol Biol. 2021. PMID: 33524413 Free PMC article.
-
Structural basis for the hydrolysis of ATP by a nucleotide binding subunit of an amino acid ABC transporter from Thermus thermophilus.J Struct Biol. 2015 Jun;190(3):367-72. doi: 10.1016/j.jsb.2015.04.012. Epub 2015 Apr 24. J Struct Biol. 2015. PMID: 25916755
-
Structural basis for allosteric cross-talk between the asymmetric nucleotide binding sites of a heterodimeric ABC exporter.Proc Natl Acad Sci U S A. 2014 Jul 29;111(30):11025-30. doi: 10.1073/pnas.1400485111. Epub 2014 Jul 16. Proc Natl Acad Sci U S A. 2014. PMID: 25030449 Free PMC article.
-
Structural basis for the mechanism of ABC transporters.Biochem Soc Trans. 2015 Oct;43(5):889-93. doi: 10.1042/BST20150047. Biochem Soc Trans. 2015. PMID: 26517899 Review.
-
The catalytic cycle of P-glycoprotein.FEBS Lett. 1995 Dec 27;377(3):285-9. doi: 10.1016/0014-5793(95)01345-8. FEBS Lett. 1995. PMID: 8549739 Review.
Cited by
-
Molecular analysis of the ribosome recycling factor ABCE1 bound to the 30S post-splitting complex.EMBO J. 2020 May 4;39(9):e103788. doi: 10.15252/embj.2019103788. Epub 2020 Feb 17. EMBO J. 2020. PMID: 32064661 Free PMC article.
-
Heliorhodopsin-mediated light-modulation of ABC transporter.Nat Commun. 2024 May 21;15(1):4306. doi: 10.1038/s41467-024-48650-1. Nat Commun. 2024. PMID: 38773114 Free PMC article.
-
Cellular Models and In Vitro Assays for the Screening of modulators of P-gp, MRP1 and BCRP.Molecules. 2017 Apr 8;22(4):600. doi: 10.3390/molecules22040600. Molecules. 2017. PMID: 28397762 Free PMC article. Review.
-
Mechanistic diversity in ATP-binding cassette (ABC) transporters.Nat Struct Mol Biol. 2016 Jun 7;23(6):487-93. doi: 10.1038/nsmb.3216. Nat Struct Mol Biol. 2016. PMID: 27273632 Review.
-
A single power stroke by ATP binding drives substrate translocation in a heterodimeric ABC transporter.Elife. 2020 Apr 21;9:e55943. doi: 10.7554/eLife.55943. Elife. 2020. PMID: 32314962 Free PMC article.
References
Publication types
MeSH terms
Substances
LinkOut - more resources
Full Text Sources
Miscellaneous