RNA sequences and structures required for the recruitment and activity of the dengue virus polymerase
- PMID: 21183683
- PMCID: PMC3044948
- DOI: 10.1074/jbc.M110.162289
RNA sequences and structures required for the recruitment and activity of the dengue virus polymerase
Abstract
Dengue virus RNA-dependent RNA polymerase specifically binds to the viral genome by interacting with a promoter element known as stem-loop A (SLA). Although a great deal has been learned in recent years about the function of this promoter in dengue virus-infected cells, the molecular details that explain how the SLA interacts with the polymerase to promote viral RNA synthesis remain poorly understood. Using RNA binding and polymerase activity assays, we defined two elements of the SLA that are involved in polymerase interaction and RNA synthesis. Mutations at the top of the SLA resulted in RNAs that retained the ability to bind the polymerase but impaired promoter-dependent RNA synthesis. These results indicate that protein binding to the SLA is not sufficient to induce polymerase activity and that specific nucleotides of the SLA are necessary to render an active polymerase-promoter complex for RNA synthesis. We also report that protein binding to the viral RNA induces conformational changes downstream of the promoter element. Furthermore, we found that structured RNA elements at the 3' end of the template repress dengue virus polymerase activity in the context of a fully active SLA promoter. Using assays to evaluate initiation of RNA synthesis at the viral 3'-UTR, we found that the RNA-RNA interaction mediated by 5'-3'-hybridization was able to release the silencing effect of the 3'-stem-loop structure. We propose that the long range RNA-RNA interactions in the viral genome play multiple roles during RNA synthesis. Together, we provide new molecular details about the promoter-dependent dengue virus RNA polymerase activity.
Figures
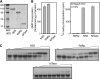
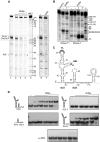
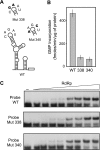
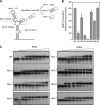
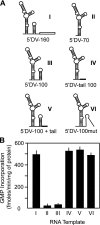
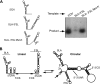
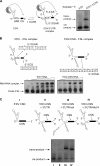
Similar articles
-
The F1 motif of dengue virus polymerase NS5 is involved in promoter-dependent RNA synthesis.J Virol. 2011 Jun;85(12):5745-56. doi: 10.1128/JVI.02343-10. Epub 2011 Apr 6. J Virol. 2011. PMID: 21471248 Free PMC article.
-
Characterization of SLA RNA promoter from dengue virus and its interaction with the viral non-structural NS5 protein.Biochimie. 2024 Jul;222:87-100. doi: 10.1016/j.biochi.2024.02.005. Epub 2024 Feb 24. Biochimie. 2024. PMID: 38408720
-
Interactions between the Dengue Virus Polymerase NS5 and Stem-Loop A.J Virol. 2017 May 12;91(11):e00047-17. doi: 10.1128/JVI.00047-17. Print 2017 Jun 1. J Virol. 2017. PMID: 28356528 Free PMC article.
-
Functional RNA elements in the dengue virus genome.Viruses. 2011 Sep;3(9):1739-56. doi: 10.3390/v3091739. Epub 2011 Sep 15. Viruses. 2011. PMID: 21994804 Free PMC article. Review.
-
Structure and function in promoter escape by T7 RNA polymerase.Prog Nucleic Acid Res Mol Biol. 2005;80:323-47. doi: 10.1016/S0079-6603(05)80008-X. Prog Nucleic Acid Res Mol Biol. 2005. PMID: 16164978 Review. No abstract available.
Cited by
-
Core protein-mediated 5'-3' annealing of the West Nile virus genomic RNA in vitro.Virus Res. 2012 Aug;167(2):226-35. doi: 10.1016/j.virusres.2012.05.003. Epub 2012 May 28. Virus Res. 2012. PMID: 22652509 Free PMC article.
-
Novel ATP-independent RNA annealing activity of the dengue virus NS3 helicase.PLoS One. 2012;7(4):e36244. doi: 10.1371/journal.pone.0036244. Epub 2012 Apr 30. PLoS One. 2012. PMID: 22558403 Free PMC article.
-
A Proline-Rich N-Terminal Region of the Dengue Virus NS3 Is Crucial for Infectious Particle Production.J Virol. 2016 May 12;90(11):5451-61. doi: 10.1128/JVI.00206-16. Print 2016 Jun 1. J Virol. 2016. PMID: 27009958 Free PMC article.
-
Structures of flavivirus RNA promoters suggest two binding modes with NS5 polymerase.Nat Commun. 2021 May 5;12(1):2530. doi: 10.1038/s41467-021-22846-1. Nat Commun. 2021. PMID: 33953197 Free PMC article.
-
Flavivirus RNA-Dependent RNA Polymerase Interacts with Genome UTRs and Viral Proteins to Facilitate Flavivirus RNA Replication.Viruses. 2019 Oct 10;11(10):929. doi: 10.3390/v11100929. Viruses. 2019. PMID: 31658680 Free PMC article. Review.
References
-
- Gubler D., Kuno G., Markoff L. (2007) in Fields Virology, Vol. 1, pp. 1153–1252, Lippincott-Raven, Philadelphia
-
- Gamarnik A. V. (2010) in Frontiers in Dengue Virus Reserach (Hanley K. A., Weaver S. C. eds) pp. 55–78, Caister Academic Press, Norfolk, UK
-
- Guzmán Tirado M. G. (1980) Rev. Cubana Med. Trop. 32, 123–130 - PubMed
-
- Gritsun T. S., Gould E. A. (2007) Virology 366, 8–15 - PubMed
Publication types
MeSH terms
Substances
Grants and funding
LinkOut - more resources
Full Text Sources