Intraphagosomal peroxynitrite as a macrophage-derived cytotoxin against internalized Trypanosoma cruzi: consequences for oxidative killing and role of microbial peroxiredoxins in infectivity
- PMID: 21098483
- PMCID: PMC3057850
- DOI: 10.1074/jbc.M110.167247
Intraphagosomal peroxynitrite as a macrophage-derived cytotoxin against internalized Trypanosoma cruzi: consequences for oxidative killing and role of microbial peroxiredoxins in infectivity
Abstract
Macrophage-derived radicals generated by the NADPH oxidase complex and inducible nitric-oxide synthase (iNOS) participate in cytotoxic mechanisms against microorganisms. Nitric oxide ((•)NO) plays a central role in the control of acute infection by Trypanosoma cruzi, the causative agent of Chagas disease, and we have proposed that much of its action relies on macrophage-derived peroxynitrite (ONOO(-) + ONOOH) formation, a strong oxidant arising from the reaction of (•)NO with superoxide radical (O(2)(-)). Herein, we have shown that internalization of T. cruzi trypomastigotes by macrophages triggers the assembly of the NADPH oxidase complex to yield O(2)(-) during a 60-90-min period. This does not interfere with IFN-γ-dependent iNOS induction and a sustained (•)NO production (∼24 h). The major mechanism for infection control via reactive species formation occurred when (•)NO and O(2)() were produced simultaneously, generating intraphagosomal peroxynitrite levels compatible with microbial killing. Moreover, biochemical and ultrastructural analysis confirmed cellular oxidative damage and morphological disruption in internalized parasites. Overexpression of cytosolic tryparedoxin peroxidase in T. cruzi neutralized macrophage-derived peroxynitrite-dependent cytotoxicity to parasites and favored the infection in an animal model. Collectively, the data provide, for the first time, direct support for the action of peroxynitrite as an intraphagosomal cytotoxin against pathogens and the premise that microbial peroxiredoxins facilitate infectivity via decomposition of macrophage-derived peroxynitrite.
Figures
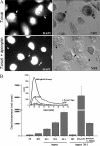
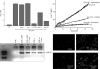
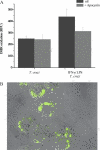
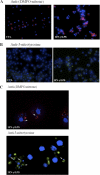
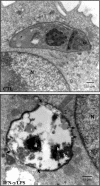
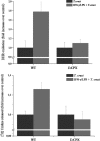
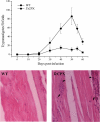
Similar articles
-
Peroxiredoxins play a major role in protecting Trypanosoma cruzi against macrophage- and endogenously-derived peroxynitrite.Biochem J. 2008 Mar 1;410(2):359-68. doi: 10.1042/BJ20071138. Biochem J. 2008. PMID: 17973627 Free PMC article.
-
Nitric oxide diffusion to red blood cells limits extracellular, but not intraphagosomal, peroxynitrite formation by macrophages.Free Radic Biol Med. 2015 Oct;87:346-55. doi: 10.1016/j.freeradbiomed.2015.06.027. Epub 2015 Jun 26. Free Radic Biol Med. 2015. PMID: 26119787
-
Cytosolic Fe-superoxide dismutase safeguards Trypanosoma cruzi from macrophage-derived superoxide radical.Proc Natl Acad Sci U S A. 2019 Apr 30;116(18):8879-8888. doi: 10.1073/pnas.1821487116. Epub 2019 Apr 12. Proc Natl Acad Sci U S A. 2019. PMID: 30979807 Free PMC article.
-
The effects of nitric oxide on the immune system during Trypanosoma cruzi infection.Mem Inst Oswaldo Cruz. 2009 Jul;104 Suppl 1:236-45. doi: 10.1590/s0074-02762009000900030. Mem Inst Oswaldo Cruz. 2009. PMID: 19753479 Review.
-
Myeloid-Derived Suppressor Cells in Trypanosoma cruzi Infection.Front Cell Infect Microbiol. 2021 Aug 27;11:737364. doi: 10.3389/fcimb.2021.737364. eCollection 2021. Front Cell Infect Microbiol. 2021. PMID: 34513737 Free PMC article. Review.
Cited by
-
Immuno-spin trapping from biochemistry to medicine: advances, challenges, and pitfalls. Focus on protein-centered radicals.Biochim Biophys Acta. 2014 Feb;1840(2):722-9. doi: 10.1016/j.bbagen.2013.04.039. Epub 2013 May 2. Biochim Biophys Acta. 2014. PMID: 23644035 Free PMC article. Review.
-
Peroxynitrite, a stealthy biological oxidant.J Biol Chem. 2013 Sep 13;288(37):26464-72. doi: 10.1074/jbc.R113.472936. Epub 2013 Jul 16. J Biol Chem. 2013. PMID: 23861390 Free PMC article. Review.
-
Synergistic effects of oxidative and acid stress on bacterial membranes of Escherichia coli and Staphylococcus simulans.Commun Biol. 2024 Sep 17;7(1):1161. doi: 10.1038/s42003-024-06862-7. Commun Biol. 2024. PMID: 39289481 Free PMC article.
-
Mycothiol/mycoredoxin 1-dependent reduction of the peroxiredoxin AhpE from Mycobacterium tuberculosis.J Biol Chem. 2014 Feb 21;289(8):5228-39. doi: 10.1074/jbc.M113.510248. Epub 2013 Dec 30. J Biol Chem. 2014. PMID: 24379404 Free PMC article.
-
The nitric oxide donor, (Z)-1-[N-(2-aminoethyl)-N-(2-ammonioethyl)amino]diazen-1-ium-1,2-diolate (DETA-NONOate/D-NO), increases survival by attenuating hyperoxia-compromised innate immunity in bacterial clearance in a mouse model of ventilator-associated pneumonia.Biochem Pharmacol. 2020 Jun;176:113817. doi: 10.1016/j.bcp.2020.113817. Epub 2020 Jan 20. Biochem Pharmacol. 2020. PMID: 31972169 Free PMC article.
References
Publication types
MeSH terms
Substances
Grants and funding
LinkOut - more resources
Full Text Sources