Arabidopsis VILLIN5, an actin filament bundling and severing protein, is necessary for normal pollen tube growth
- PMID: 20807879
- PMCID: PMC2947167
- DOI: 10.1105/tpc.110.076257
Arabidopsis VILLIN5, an actin filament bundling and severing protein, is necessary for normal pollen tube growth
Abstract
A dynamic actin cytoskeleton is essential for pollen germination and tube growth. However, the molecular mechanisms underlying the organization and turnover of the actin cytoskeleton in pollen remain poorly understood. Villin plays a key role in the formation of higher-order structures from actin filaments and in the regulation of actin dynamics in eukaryotic cells. It belongs to the villin/gelsolin/fragmin superfamily of actin binding proteins and is composed of six gelsolin-homology domains at its core and a villin headpiece domain at its C terminus. Recently, several villin family members from plants have been shown to sever, cap, and bundle actin filaments in vitro. Here, we characterized a villin isovariant, Arabidopsis thaliana VILLIN5 (VLN5), that is highly and preferentially expressed in pollen. VLN5 loss-of-function retarded pollen tube growth and sensitized actin filaments in pollen grains and tubes to latrunculin B. In vitro biochemical analyses revealed that VLN5 is a typical member of the villin family and retains a full suite of activities, including barbed-end capping, filament bundling, and calcium-dependent severing. The severing activity was confirmed with time-lapse evanescent wave microscopy of individual actin filaments in vitro. We propose that VLN5 is a major regulator of actin filament stability and turnover that functions in concert with oscillatory calcium gradients in pollen and therefore plays an integral role in pollen germination and tube growth.
Figures
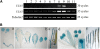
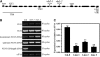
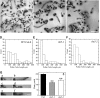
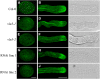
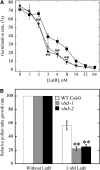
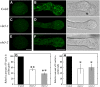
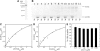
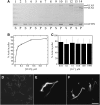
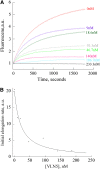
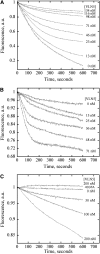
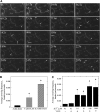
Similar articles
-
Arabidopsis villins promote actin turnover at pollen tube tips and facilitate the construction of actin collars.Plant Cell. 2013 May;25(5):1803-17. doi: 10.1105/tpc.113.110940. Epub 2013 May 28. Plant Cell. 2013. PMID: 23715472 Free PMC article.
-
Arabidopsis VILLIN5 bundles actin filaments using a novel mechanism.Plant J. 2024 Sep;119(6):2854-2866. doi: 10.1111/tpj.16956. Epub 2024 Aug 2. Plant J. 2024. PMID: 39093617
-
Arabidopsis VILLIN1 generates actin filament cables that are resistant to depolymerization.Plant Cell. 2005 Feb;17(2):486-501. doi: 10.1105/tpc.104.028555. Epub 2005 Jan 19. Plant Cell. 2005. PMID: 15659626 Free PMC article.
-
Plant villins: versatile actin regulatory proteins.J Integr Plant Biol. 2015 Jan;57(1):40-9. doi: 10.1111/jipb.12293. Epub 2014 Dec 17. J Integr Plant Biol. 2015. PMID: 25294278 Review.
-
Actin dynamics in the cortical array of plant cells.Curr Opin Plant Biol. 2013 Dec;16(6):678-87. doi: 10.1016/j.pbi.2013.10.012. Epub 2013 Nov 15. Curr Opin Plant Biol. 2013. PMID: 24246228 Review.
Cited by
-
Arabidopsis vacuolar H+-ATPase (V-ATPase) B subunits are involved in actin cytoskeleton remodeling via binding to, bundling, and stabilizing F-actin.J Biol Chem. 2012 Jun 1;287(23):19008-17. doi: 10.1074/jbc.M111.281873. Epub 2012 Feb 27. J Biol Chem. 2012. PMID: 22371505 Free PMC article.
-
Cadmium toxicity: effects on cytoskeleton, vesicular trafficking and cell wall construction.Plant Signal Behav. 2012 Mar;7(3):345-8. doi: 10.4161/psb.18992. Epub 2012 Mar 1. Plant Signal Behav. 2012. PMID: 22499203 Free PMC article. Review.
-
Arabidopsis RIC1 Severs Actin Filaments at the Apex to Regulate Pollen Tube Growth.Plant Cell. 2015 Apr;27(4):1140-61. doi: 10.1105/tpc.114.135400. Epub 2015 Mar 24. Plant Cell. 2015. PMID: 25804540 Free PMC article.
-
Arabidopsis FIM5 decorates apical actin filaments and regulates their organization in the pollen tube.J Exp Bot. 2016 May;67(11):3407-17. doi: 10.1093/jxb/erw160. Epub 2016 Apr 25. J Exp Bot. 2016. PMID: 27117336 Free PMC article.
-
A SAC Phosphoinositide Phosphatase Controls Rice Development via Hydrolyzing PI4P and PI(4,5)P2.Plant Physiol. 2020 Mar;182(3):1346-1358. doi: 10.1104/pp.19.01131. Epub 2019 Dec 27. Plant Physiol. 2020. PMID: 31882455 Free PMC article.
References
-
- Andrianantoandro E., Pollard T.D. (2006). Mechanism of actin filament turnover by severing and nucleation at different concentrations of ADF/cofilin. Mol. Cell 24: 13–23 - PubMed
-
- Blanchoin L., Amann K.J., Higgs H.N., Marchand J.B., Kaiser D.A., Pollard T.D. (2000). Direct observation of dendritic actin filament networks nucleated by Arp2/3 complex and WASP/Scar proteins. Nature 404: 1007–1011 - PubMed
Publication types
MeSH terms
Substances
LinkOut - more resources
Full Text Sources
Molecular Biology Databases
Research Materials
Miscellaneous