Three-dimensional optical method for integrated visualization of mouse islet microstructure and vascular network with subcellular-level resolution
- PMID: 20799820
- PMCID: PMC3188637
- DOI: 10.1117/1.3470241
Three-dimensional optical method for integrated visualization of mouse islet microstructure and vascular network with subcellular-level resolution
Abstract
Microscopic visualization of islets of Langerhans under normal and diabetic conditions is essential for understanding the pathophysiology of the disease. The intrinsic opacity of pancreata, however, limits optical accessibility for high-resolution light microscopy of islets in situ. Because the standard microtome-based, 2-D tissue analysis confines visualization of the islet architecture at a specific cut plane, 3-D representation of image data is preferable for islet assessment. We applied optical clearing to minimize the random light scattering in the mouse pancreatic tissue. The optical-cleared pancreas allowed penetrative, 3-D microscopic imaging of the islet microstructure and vasculature. Specifically, the islet vasculature was revealed by vessel painting-lipophilic dye labeling of blood vessels-for confocal microscopy. The voxel-based confocal micrographs were digitally processed with projection algorithms for 3-D visualization. Unlike the microtome-based tissue imaging, this optical method for penetrative imaging of mouse islets yielded clear, continuous optical sections for an integrated visualization of the islet microstructure and vasculature with subcellular-level resolution. We thus provide a useful imaging approach to change our conventional planar view of the islet structure into a 3-D panorama for better understanding of the islet physiology.
Figures
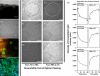
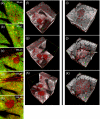
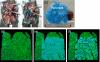
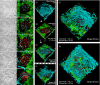
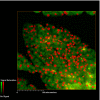
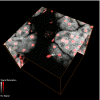
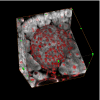
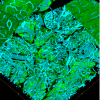
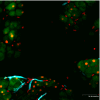
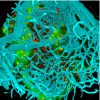
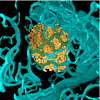
Similar articles
-
Optical clearing facilitates integrated 3D visualization of mouse ileal microstructure and vascular network with high definition.Microvasc Res. 2010 Dec;80(3):512-21. doi: 10.1016/j.mvr.2010.06.003. Epub 2010 Jun 18. Microvasc Res. 2010. PMID: 20600164
-
Microtome-free 3-dimensional confocal imaging method for visualization of mouse intestine with subcellular-level resolution.Gastroenterology. 2009 Aug;137(2):453-65. doi: 10.1053/j.gastro.2009.05.008. Epub 2009 May 15. Gastroenterology. 2009. PMID: 19447107 Free PMC article.
-
Three-dimensional analysis of the islet vasculature.Anat Rec (Hoboken). 2012 Sep;295(9):1473-81. doi: 10.1002/ar.22530. Epub 2012 Jul 16. Anat Rec (Hoboken). 2012. PMID: 22807267
-
Imaging of the islet neural network.Diabetes Obes Metab. 2014 Sep;16 Suppl 1:77-86. doi: 10.1111/dom.12342. Diabetes Obes Metab. 2014. PMID: 25200300 Review.
-
Pancreas Optical Clearing and 3-D Microscopy in Health and Diabetes.Front Endocrinol (Lausanne). 2021 Apr 26;12:644826. doi: 10.3389/fendo.2021.644826. eCollection 2021. Front Endocrinol (Lausanne). 2021. PMID: 33981285 Free PMC article. Review.
Cited by
-
3-D imaging of islets in obesity: formation of the islet-duct complex and neurovascular remodeling in young hyperphagic mice.Int J Obes (Lond). 2016 Apr;40(4):685-97. doi: 10.1038/ijo.2015.224. Epub 2015 Oct 26. Int J Obes (Lond). 2016. PMID: 26499436
-
3-D imaging and illustration of the perfusive mouse islet sympathetic innervation and its remodelling in injury.Diabetologia. 2012 Dec;55(12):3252-61. doi: 10.1007/s00125-012-2699-6. Epub 2012 Aug 30. Diabetologia. 2012. PMID: 22930160
-
Multiscale and multimodal imaging for three-dimensional vascular and histomorphological organ structure analysis of the pancreas.Sci Rep. 2024 May 2;14(1):10136. doi: 10.1038/s41598-024-60254-9. Sci Rep. 2024. PMID: 38698049 Free PMC article.
-
Feasibility of a hybrid elastographic-microfluidic device to rapidly process and assess pancreatic cancer biopsies for pathologists.Health Innov Point Care Conf. 2014 Oct;2014:271-275. doi: 10.1109/HIC.2014.7038927. Health Innov Point Care Conf. 2014. PMID: 26110186 Free PMC article.
-
3-D Imaging Reveals Participation of Donor Islet Schwann Cells and Pericytes in Islet Transplantation and Graft Neurovascular Regeneration.EBioMedicine. 2015 Jan 27;2(2):109-19. doi: 10.1016/j.ebiom.2015.01.014. eCollection 2015 Feb. EBioMedicine. 2015. PMID: 26137552 Free PMC article.
References
-
- Brunicardi F. C., Stagner J., Bonner-Weir S., Wayland H., Kleinman R., Livingston E., Guth P., Menger M., McCuskey R., Intaglietta M., Charles A., Ashley S., Cheung A., Ipp E., Gilman S., Howard T., and Passaro E., “Microcirculation of the islets of Langerhans—Long Beach Veterans Administration Regional Medical Education Center Symposium,” Diabetes DIAEAZ 45(4), 385–392 (1996). - PubMed
-
- Tuchin V. V., “A clear vision for laser diagnostics,” IEEE J. Sel. Top. Quantum Electron. IJSQEN 13(6), 1621–1628 (2007).10.1109/JSTQE.2007.911313 - DOI
Publication types
MeSH terms
Grants and funding
LinkOut - more resources
Full Text Sources
Other Literature Sources