Reduction of cysteine sulfinic acid in eukaryotic, typical 2-Cys peroxiredoxins by sulfiredoxin
- PMID: 20712415
- PMCID: PMC3110103
- DOI: 10.1089/ars.2010.3564
Reduction of cysteine sulfinic acid in eukaryotic, typical 2-Cys peroxiredoxins by sulfiredoxin
Abstract
The eukaryotic, typical 2-Cys peroxiredoxins (Prxs) are inactivated by hyperoxidation of one of their active-site cysteine residues to cysteine sulfinic acid. This covalent modification is thought to enable hydrogen peroxide-mediated cell signaling and to act as a functional switch between a peroxidase and a high-molecular-weight chaperone. Moreover, hyperoxidation has been implicated in a variety of disease states associated with oxidative stress, including cancer and aging-associated pathologies. A repair enzyme, sulfiredoxin (Srx), reduces the sulfinic acid moiety by using an unusual ATP-dependent mechanism. In this process, the Prx molecule undergoes dramatic structural rearrangements to facilitate repair. Structural, kinetic, mutational, and mass spectrometry-based approaches have been used to dissect the molecular basis for Srx catalysis. The available data support the direct formation of Cys sulfinic acid phosphoryl ester and protein-based thiosulfinate intermediates. This review discusses the role of Srx in the reversal of Prx hyperoxidation, the questions raised concerning the reductant required for human Srx regeneration, and the deglutathionylating activity of Srx. The complex interplay between Prx hyperoxidation, other forms of Prx covalent modification, and the oligomeric state also are discussed.
Figures
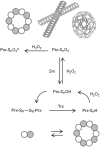
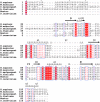
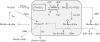
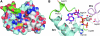
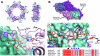
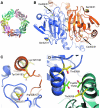
Similar articles
-
Sulfiredoxin, the cysteine sulfinic acid reductase specific to 2-Cys peroxiredoxin: its discovery, mechanism of action, and biological significance.Kidney Int Suppl. 2007 Aug;(106):S3-8. doi: 10.1038/sj.ki.5002380. Kidney Int Suppl. 2007. PMID: 17653208 Review.
-
Identification of intact protein thiosulfinate intermediate in the reduction of cysteine sulfinic acid in peroxiredoxin by human sulfiredoxin.J Biol Chem. 2008 Aug 22;283(34):22890-4. doi: 10.1074/jbc.C800124200. Epub 2008 Jun 30. J Biol Chem. 2008. PMID: 18593714 Free PMC article.
-
Reduction of cysteine sulfinic acid in peroxiredoxin by sulfiredoxin proceeds directly through a sulfinic phosphoryl ester intermediate.J Biol Chem. 2008 Aug 29;283(35):23846-51. doi: 10.1074/jbc.M803244200. Epub 2008 Jun 24. J Biol Chem. 2008. PMID: 18579529 Free PMC article.
-
Reduction of cysteine sulfinic acid by sulfiredoxin is specific to 2-cys peroxiredoxins.J Biol Chem. 2005 Feb 4;280(5):3125-8. doi: 10.1074/jbc.C400496200. Epub 2004 Dec 8. J Biol Chem. 2005. PMID: 15590625
-
Role of sulfiredoxin as a regulator of peroxiredoxin function and regulation of its expression.Free Radic Biol Med. 2012 Aug 1;53(3):447-56. doi: 10.1016/j.freeradbiomed.2012.05.020. Epub 2012 May 24. Free Radic Biol Med. 2012. PMID: 22634055 Review.
Cited by
-
Hyperoxidation of Peroxiredoxins and Effects on Physiology of Drosophila.Antioxidants (Basel). 2021 Apr 15;10(4):606. doi: 10.3390/antiox10040606. Antioxidants (Basel). 2021. PMID: 33920774 Free PMC article.
-
Kinetic modeling of H2O2 dynamics in the mitochondria of HeLa cells.PLoS Comput Biol. 2020 Sep 14;16(9):e1008202. doi: 10.1371/journal.pcbi.1008202. eCollection 2020 Sep. PLoS Comput Biol. 2020. PMID: 32925922 Free PMC article.
-
Chemoselective ligation of sulfinic acids with aryl-nitroso compounds.Angew Chem Int Ed Engl. 2012 Jun 25;51(26):6502-5. doi: 10.1002/anie.201201812. Epub 2012 May 29. Angew Chem Int Ed Engl. 2012. PMID: 22644884 Free PMC article.
-
Differential parameters between cytosolic 2-Cys peroxiredoxins, PRDX1 and PRDX2.Protein Sci. 2019 Jan;28(1):191-201. doi: 10.1002/pro.3520. Epub 2018 Nov 12. Protein Sci. 2019. PMID: 30284335 Free PMC article.
-
Destroy and exploit: catalyzed removal of hydroperoxides from the endoplasmic reticulum.Int J Cell Biol. 2013;2013:180906. doi: 10.1155/2013/180906. Epub 2013 Oct 24. Int J Cell Biol. 2013. PMID: 24282412 Free PMC article. Review.
References
-
- Abbas K. Breton J. Drapier JC. The interplay between nitric oxide and peroxiredoxins. Immunobiology. 2008;213:815–822. - PubMed
-
- Aran M. Ferrero DS. Pagano E. Wolosiuk RA. Typical 2-Cys peroxiredoxins: modulation by covalent transformations and noncovalent interactions. FEBS J. 2009;276:2478–2493. - PubMed
-
- Avellini C. Baccarani U. Trevisan G. Cesaratto L. Vascotto C. D'Aurizio F. Pandolfi M. Adani GL. Tell G. Redox proteomics and immunohistology to study molecular events during ischemia-reperfusion in human liver. Transplant Proc. 2007;39:1755–1760. - PubMed
-
- Barranco-Medina S. Lazaro JJ. Dietz KJ. The oligomeric conformation of peroxiredoxins links redox state to function. FEBS Lett. 2009;583:1809–1816. - PubMed
-
- Basu MK. Koonin EV. Evolution of eukaryotic cysteine sulfinic acid reductase, sulfiredoxin (Srx), from bacterial chromosome partitioning protein ParB. Cell Cycle. 2005;4:947–952. - PubMed
Publication types
MeSH terms
Substances
Grants and funding
LinkOut - more resources
Full Text Sources