From the Cover: Charge interactions can dominate the dimensions of intrinsically disordered proteins
- PMID: 20639465
- PMCID: PMC2930438
- DOI: 10.1073/pnas.1001743107
From the Cover: Charge interactions can dominate the dimensions of intrinsically disordered proteins
Erratum in
- Proc Natl Acad Sci U S A. 2013 Oct 8;110(41):16693
Abstract
Many eukaryotic proteins are disordered under physiological conditions, and fold into ordered structures only on binding to their cellular targets. Such intrinsically disordered proteins (IDPs) often contain a large fraction of charged amino acids. Here, we use single-molecule Förster resonance energy transfer to investigate the influence of charged residues on the dimensions of unfolded and intrinsically disordered proteins. We find that, in contrast to the compact unfolded conformations that have been observed for many proteins at low denaturant concentration, IDPs can exhibit a prominent expansion at low ionic strength that correlates with their net charge. Charge-balanced polypeptides, however, can exhibit an additional collapse at low ionic strength, as predicted by polyampholyte theory from the attraction between opposite charges in the chain. The pronounced effect of charges on the dimensions of unfolded proteins has important implications for the cellular functions of IDPs.
Conflict of interest statement
The authors declare no conflict of interest.
Figures
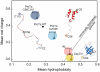
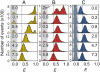
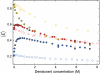
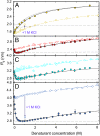
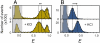
Comment in
-
To fold or expand--a charged question.Proc Natl Acad Sci U S A. 2010 Aug 17;107(33):14519-20. doi: 10.1073/pnas.1008673107. Epub 2010 Aug 3. Proc Natl Acad Sci U S A. 2010. PMID: 20682745 Free PMC article. No abstract available.
Similar articles
-
To fold or expand--a charged question.Proc Natl Acad Sci U S A. 2010 Aug 17;107(33):14519-20. doi: 10.1073/pnas.1008673107. Epub 2010 Aug 3. Proc Natl Acad Sci U S A. 2010. PMID: 20682745 Free PMC article. No abstract available.
-
Single-molecule fluorescence studies of intrinsically disordered proteins.Methods Enzymol. 2010;472:179-204. doi: 10.1016/S0076-6879(10)72010-3. Methods Enzymol. 2010. PMID: 20580965
-
Kinetics of intramolecular contact formation in a denatured protein.J Mol Biol. 2003 Sep 5;332(1):9-12. doi: 10.1016/s0022-2836(03)00891-x. J Mol Biol. 2003. PMID: 12946342
-
Understanding disordered and unfolded proteins using single-molecule FRET and polymer theory.Methods Appl Fluoresc. 2016 Nov 17;4(4):042003. doi: 10.1088/2050-6120/4/4/042003. Methods Appl Fluoresc. 2016. PMID: 28192291 Review.
-
Intrinsically disordered proteins in human diseases: introducing the D2 concept.Annu Rev Biophys. 2008;37:215-46. doi: 10.1146/annurev.biophys.37.032807.125924. Annu Rev Biophys. 2008. PMID: 18573080 Review.
Cited by
-
Quantifying the topography of the intrinsic energy landscape of flexible biomolecular recognition.Proc Natl Acad Sci U S A. 2013 Jun 25;110(26):E2342-51. doi: 10.1073/pnas.1220699110. Epub 2013 Jun 10. Proc Natl Acad Sci U S A. 2013. PMID: 23754431 Free PMC article.
-
NMR Provides Unique Insight into the Functional Dynamics and Interactions of Intrinsically Disordered Proteins.Chem Rev. 2022 May 25;122(10):9331-9356. doi: 10.1021/acs.chemrev.1c01023. Epub 2022 Apr 21. Chem Rev. 2022. PMID: 35446534 Free PMC article. Review.
-
Translocation, Rejection and Trapping of Polyampholytes.Polymers (Basel). 2022 Feb 18;14(4):797. doi: 10.3390/polym14040797. Polymers (Basel). 2022. PMID: 35215709 Free PMC article.
-
Conformational Analysis of Charged Homo-Polypeptides.Biomolecules. 2023 Feb 15;13(2):363. doi: 10.3390/biom13020363. Biomolecules. 2023. PMID: 36830732 Free PMC article.
-
Investigation of intramolecular dynamics and conformations of α-, β- and γ-synuclein.PLoS One. 2014 Jan 28;9(1):e86983. doi: 10.1371/journal.pone.0086983. eCollection 2014. PLoS One. 2014. PMID: 24489820 Free PMC article.
References
-
- Dyson HJ, Wright PE. Intrinsically unstructured proteins and their functions. Nat Rev Mol Cell Biol. 2005;6:197–208. - PubMed
-
- Dunker AK, Silman I, Uversky VN, Sussman JL. Function and structure of inherently disordered proteins. Curr Opin Struct Biol. 2008;18:756–764. - PubMed
-
- Uversky VN, Oldfield CJ, Dunker AK. Intrinsically disordered proteins in human diseases: Introducing the D2 concept. Ann Rev Biophys. 2008;37:215–246. - PubMed
Publication types
MeSH terms
Substances
LinkOut - more resources
Full Text Sources
Other Literature Sources