Review series: The cell biology of hearing
- PMID: 20624897
- PMCID: PMC2911669
- DOI: 10.1083/jcb.201001138
Review series: The cell biology of hearing
Abstract
Mammals have an astonishing ability to sense and discriminate sounds of different frequencies and intensities. Fundamental for this process are mechanosensory hair cells in the inner ear that convert sound-induced vibrations into electrical signals. The study of genes that are linked to deafness has provided insights into the cell biological mechanisms that control hair cell development and their function as mechanosensors.
Figures
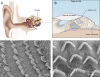
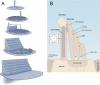
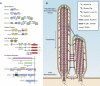
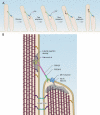
Similar articles
-
Tmc proteins are essential for zebrafish hearing where Tmc1 is not obligatory.Hum Mol Genet. 2020 Jul 29;29(12):2004-2021. doi: 10.1093/hmg/ddaa045. Hum Mol Genet. 2020. PMID: 32167554 Free PMC article.
-
[Molecular biology of hearing].Laryngorhinootologie. 2011 Mar;90 Suppl 1:S22-34. doi: 10.1055/s-0030-1270444. Epub 2011 Apr 26. Laryngorhinootologie. 2011. PMID: 21523631 Review. German.
-
Hair cells, plasma membrane Ca²⁺ ATPase and deafness.Int J Biochem Cell Biol. 2012 May;44(5):679-83. doi: 10.1016/j.biocel.2012.02.006. Epub 2012 Feb 13. Int J Biochem Cell Biol. 2012. PMID: 22349217 Review.
-
Kiaa1024L/Minar2 is essential for hearing by regulating cholesterol distribution in hair bundles.Elife. 2022 Nov 1;11:e80865. doi: 10.7554/eLife.80865. Elife. 2022. PMID: 36317962 Free PMC article.
-
Actin-bundling protein TRIOBP forms resilient rootlets of hair cell stereocilia essential for hearing.Cell. 2010 May 28;141(5):786-98. doi: 10.1016/j.cell.2010.03.049. Cell. 2010. PMID: 20510926 Free PMC article.
Cited by
-
Developmental and architectural principles of the lateral-line neural map.Front Neural Circuits. 2013 Mar 26;7:47. doi: 10.3389/fncir.2013.00047. eCollection 2013. Front Neural Circuits. 2013. PMID: 23532704 Free PMC article. Review.
-
The Cell Adhesion Molecules Roughest, Hibris, Kin of Irre and Sticks and Stones Are Required for Long Range Spacing of the Drosophila Wing Disc Sensory Sensilla.PLoS One. 2015 Jun 8;10(6):e0128490. doi: 10.1371/journal.pone.0128490. eCollection 2015. PLoS One. 2015. PMID: 26053791 Free PMC article.
-
Constitutive activation of DIA1 (DIAPH1) via C-terminal truncation causes human sensorineural hearing loss.EMBO Mol Med. 2016 Nov 2;8(11):1310-1324. doi: 10.15252/emmm.201606609. Print 2016 Nov. EMBO Mol Med. 2016. PMID: 27707755 Free PMC article.
-
Molecular biology of hearing.GMS Curr Top Otorhinolaryngol Head Neck Surg. 2011;10:Doc06. doi: 10.3205/cto000079. Epub 2012 Apr 26. GMS Curr Top Otorhinolaryngol Head Neck Surg. 2011. PMID: 22558056 Free PMC article.
-
The Emerging Role of Sperm-Associated Antigen 6 Gene in the Microtubule Function of Cells and Cancer.Mol Ther Oncolytics. 2019 Sep 10;15:101-107. doi: 10.1016/j.omto.2019.08.011. eCollection 2019 Dec 20. Mol Ther Oncolytics. 2019. PMID: 31660426 Free PMC article. Review.
References
-
- Adato A., Lefèvre G., Delprat B., Michel V., Michalski N., Chardenoux S., Weil D., El-Amraoui A., Petit C. 2005a. Usherin, the defective protein in Usher syndrome type IIA, is likely to be a component of interstereocilia ankle links in the inner ear sensory cells. Hum. Mol. Genet. 14:3921–3932 10.1093/hmg/ddi416 - DOI - PubMed
-
- Ahmed Z.M., Goodyear R., Riazuddin S., Lagziel A., Legan P.K., Behra M., Burgess S.M., Lilley K.S., Wilcox E.R., Riazuddin S., et al. 2006. The tip-link antigen, a protein associated with the transduction complex of sensory hair cells, is protocadherin-15. J. Neurosci. 26:7022–7034 10.1523/JNEUROSCI.1163-06.2006 - DOI - PMC - PubMed
-
- Alagramam K.N., Murcia C.L., Kwon H.Y., Pawlowski K.S., Wright C.G., Woychik R.P. 2001. The mouse Ames waltzer hearing-loss mutant is caused by mutation of Pcdh15, a novel protocadherin gene. Nat. Genet. 27:99–102 - PubMed
Publication types
MeSH terms
Grants and funding
LinkOut - more resources
Full Text Sources
Medical