Membrane budding and scission by the ESCRT machinery: it's all in the neck
- PMID: 20588296
- PMCID: PMC2922035
- DOI: 10.1038/nrm2937
Membrane budding and scission by the ESCRT machinery: it's all in the neck
Abstract
The endosomal sorting complexes required for transport (ESCRTs) catalyse one of the most unusual membrane remodelling events in cell biology. ESCRT-I and ESCRT-II direct membrane budding away from the cytosol by stabilizing bud necks without coating the buds and without being consumed in the buds. ESCRT-III cleaves the bud necks from their cytosolic faces. ESCRT-III-mediated membrane neck cleavage is crucial for many processes, including the biogenesis of multivesicular bodies, viral budding, cytokinesis and, probably, autophagy. Recent studies of ultrastructures induced by ESCRT-III overexpression in cells and the in vitro reconstitution of the budding and scission reactions have led to breakthroughs in understanding these remarkable membrane reactions.
Figures
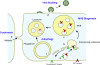
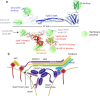
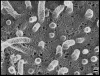
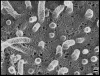
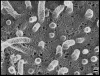
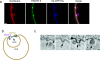
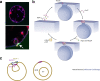
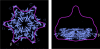
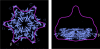
Similar articles
-
Molecular mechanism of multivesicular body biogenesis by ESCRT complexes.Nature. 2010 Apr 8;464(7290):864-9. doi: 10.1038/nature08849. Epub 2010 Mar 21. Nature. 2010. PMID: 20305637 Free PMC article.
-
ESCRT-III polymers in membrane neck constriction.Trends Cell Biol. 2012 Mar;22(3):133-40. doi: 10.1016/j.tcb.2011.11.007. Epub 2012 Jan 10. Trends Cell Biol. 2012. PMID: 22240455
-
Bro1 binding to Snf7 regulates ESCRT-III membrane scission activity in yeast.J Cell Biol. 2011 Jan 24;192(2):295-306. doi: 10.1083/jcb.201007018. J Cell Biol. 2011. PMID: 21263029 Free PMC article.
-
Dynamics of ESCRT proteins.Cell Mol Life Sci. 2012 Dec;69(24):4121-33. doi: 10.1007/s00018-012-1035-0. Epub 2012 Jun 6. Cell Mol Life Sci. 2012. PMID: 22669260 Free PMC article. Review.
-
Differential requirements of mammalian ESCRTs in multivesicular body formation, virus budding and cell division.FEBS J. 2012 Apr;279(8):1399-406. doi: 10.1111/j.1742-4658.2012.08534.x. Epub 2012 Mar 23. FEBS J. 2012. PMID: 22340600 Review.
Cited by
-
Hijacking host extracellular vesicle machinery by hepatotropic viruses: current understandings and future prospects.J Biomed Sci. 2024 Oct 5;31(1):97. doi: 10.1186/s12929-024-01063-0. J Biomed Sci. 2024. PMID: 39369194 Free PMC article. Review.
-
HIV-1 assembly, budding, and maturation.Cold Spring Harb Perspect Med. 2012 Jul;2(7):a006924. doi: 10.1101/cshperspect.a006924. Cold Spring Harb Perspect Med. 2012. PMID: 22762019 Free PMC article. Review.
-
Syndecan-syntenin-ALIX regulates the biogenesis of exosomes.Nat Cell Biol. 2012 Jun 3;14(7):677-85. doi: 10.1038/ncb2502. Nat Cell Biol. 2012. PMID: 22660413
-
Signaling-mediated control of ubiquitin ligases in endocytosis.BMC Biol. 2012 Mar 15;10:25. doi: 10.1186/1741-7007-10-25. BMC Biol. 2012. PMID: 22420864 Free PMC article. Review.
-
Shaping development with ESCRTs.Nat Cell Biol. 2011 Dec 22;14(1):38-45. doi: 10.1038/ncb2381. Nat Cell Biol. 2011. PMID: 22193162 Review.
References
-
- Gruenberg J, Stenmark H. The biogenesis of multivesicular endosomes. Nat. Rev. Mol. Cell Biol. 2004;5:317–323. - PubMed
-
- Russell MRG, Nickerson DP, Odorizzi G. Molecular mechanisms of late endosome morphology, identity and sorting. Curr. Opin. Cell Biol. 2006;18:422–428. - PubMed
-
- Peplowska K, Markgraf DF, Ostrowicz CW, Bange G, Ungermann C. The CORVET tethering complex interacts with the yeast Rab5 homolog Vps21 and is involved in endo-lysosomal biogenesis. Dev. Cell. 2007;12:739–750. - PubMed
Publication types
MeSH terms
Substances
Grants and funding
LinkOut - more resources
Full Text Sources
Other Literature Sources
Molecular Biology Databases