Identification of a coiled coil in werner syndrome protein that facilitates multimerization and promotes exonuclease processivity
- PMID: 20516064
- PMCID: PMC2919133
- DOI: 10.1074/jbc.M110.124941
Identification of a coiled coil in werner syndrome protein that facilitates multimerization and promotes exonuclease processivity
Abstract
Werner syndrome (WS) is a rare progeroid disorder characterized by genomic instability, increased cancer incidence, and early onset of a variety of aging pathologies. WS is unique among early aging syndromes in that affected individuals are developmentally normal, and phenotypic onset is in early adulthood. The protein defective in WS (WRN) is a member of the large RecQ family of helicases but is unique among this family in having an exonuclease. RecQ helicases form multimers, but the mechanism and consequence of multimerization remain incompletely defined. Here, we identify a novel heptad repeat coiled coil region between the WRN nuclease and helicase domains that facilitates multimerization of WRN. We mapped a novel and unique DNA-dependent protein kinase phosphorylation site proximal to the WRN multimerization region. However, phosphorylation at this site affected neither exonuclease activity nor multimeric state. We found that WRN nuclease is stimulated by DNA-dependent protein kinase independently of kinase activity or WRN nuclease multimeric status. In addition, WRN nuclease multimerization significantly increased nuclease processivity. We found that the novel WRN coiled coil domain is necessary for multimerization of the nuclease domain and sufficient to multimerize with full-length WRN in human cells. Importantly, correct homomultimerization is required for WRN function in vivo as overexpression of this multimerization domain caused increased sensitivity to camptothecin and 4-nitroquinoline 1-oxide similar to that in cells lacking functional WRN protein.
Figures
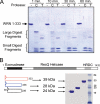
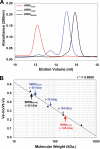
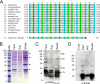
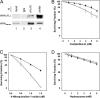
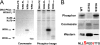
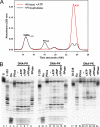
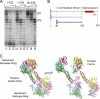
Similar articles
-
Multimerization domains are associated with apparent strand exchange activity in BLM and WRN DNA helicases.DNA Repair (Amst). 2014 Oct;22:137-46. doi: 10.1016/j.dnarep.2014.07.015. Epub 2014 Sep 3. DNA Repair (Amst). 2014. PMID: 25198671 Free PMC article.
-
A fluorescence-based exonuclease assay to characterize DmWRNexo, orthologue of human progeroid WRN exonuclease, and its application to other nucleases.J Vis Exp. 2013 Dec 23;(82):e50722. doi: 10.3791/50722. J Vis Exp. 2013. PMID: 24378758 Free PMC article.
-
Werner syndrome protein. I. DNA helicase and dna exonuclease reside on the same polypeptide.J Biol Chem. 1998 Dec 18;273(51):34139-44. doi: 10.1074/jbc.273.51.34139. J Biol Chem. 1998. PMID: 9852073
-
The Werner's Syndrome RecQ helicase/exonuclease at the nexus of cancer and aging.Hawaii Med J. 2011 Mar;70(3):52-5. Hawaii Med J. 2011. PMID: 21365542 Free PMC article. Review.
-
Role of Werner syndrome gene product helicase in carcinogenesis and in resistance to genotoxins by cancer cells.Cancer Sci. 2008 May;99(5):843-8. doi: 10.1111/j.1349-7006.2008.00778.x. Epub 2008 Feb 26. Cancer Sci. 2008. PMID: 18312465 Free PMC article. Review.
Cited by
-
Werner Syndrome Protein and DNA Replication.Int J Mol Sci. 2018 Nov 2;19(11):3442. doi: 10.3390/ijms19113442. Int J Mol Sci. 2018. PMID: 30400178 Free PMC article. Review.
-
Serines 440 and 467 in the Werner syndrome protein are phosphorylated by DNA-PK and affects its dynamics in response to DNA double strand breaks.Aging (Albany NY). 2014 Jan;6(1):70-81. doi: 10.18632/aging.100629. Aging (Albany NY). 2014. PMID: 24429382 Free PMC article.
-
Cooperative DNA binding and protein/DNA fiber formation increases the activity of the Dnmt3a DNA methyltransferase.J Biol Chem. 2014 Oct 24;289(43):29602-13. doi: 10.1074/jbc.M114.572032. Epub 2014 Aug 21. J Biol Chem. 2014. PMID: 25147181 Free PMC article.
-
Nonenzymatic role for WRN in preserving nascent DNA strands after replication stress.Cell Rep. 2014 Nov 20;9(4):1387-401. doi: 10.1016/j.celrep.2014.10.025. Epub 2014 Nov 6. Cell Rep. 2014. PMID: 25456133 Free PMC article.
-
Multimerization domains are associated with apparent strand exchange activity in BLM and WRN DNA helicases.DNA Repair (Amst). 2014 Oct;22:137-46. doi: 10.1016/j.dnarep.2014.07.015. Epub 2014 Sep 3. DNA Repair (Amst). 2014. PMID: 25198671 Free PMC article.
References
Publication types
MeSH terms
Substances
Grants and funding
LinkOut - more resources
Full Text Sources
Molecular Biology Databases