Actin-bundling protein TRIOBP forms resilient rootlets of hair cell stereocilia essential for hearing
- PMID: 20510926
- PMCID: PMC2879707
- DOI: 10.1016/j.cell.2010.03.049
Actin-bundling protein TRIOBP forms resilient rootlets of hair cell stereocilia essential for hearing
Abstract
Inner ear hair cells detect sound through deflection of mechanosensory stereocilia. Each stereocilium is supported by a paracrystalline array of parallel actin filaments that are packed more densely at the base, forming a rootlet extending into the cell body. The function of rootlets and the molecules responsible for their formation are unknown. We found that TRIOBP, a cytoskeleton-associated protein mutated in human hereditary deafness DFNB28, is localized to rootlets. In vitro, purified TRIOBP isoform 4 protein organizes actin filaments into uniquely dense bundles reminiscent of rootlets but distinct from bundles formed by espin, an actin crosslinker in stereocilia. We generated mutant Triobp mice (Triobp(Deltaex8/Deltaex8)) that are profoundly deaf. Stereocilia of Triobp(Deltaex8/Deltaex8) mice develop normally but fail to form rootlets and are easier to deflect and damage. Thus, F-actin bundling by TRIOBP provides durability and rigidity for normal mechanosensitivity of stereocilia and may contribute to resilient cytoskeletal structures elsewhere.
Copyright 2010 Elsevier Inc. All rights reserved.
Figures
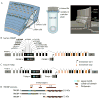
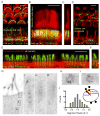
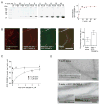
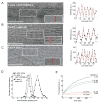
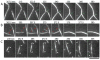
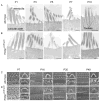
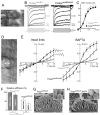
Comment in
-
Wrapping up stereocilia rootlets.Cell. 2010 May 28;141(5):748-50. doi: 10.1016/j.cell.2010.05.022. Cell. 2010. PMID: 20510920
Similar articles
-
TRIOBP-5 sculpts stereocilia rootlets and stiffens supporting cells enabling hearing.JCI Insight. 2019 Jun 20;4(12):e128561. doi: 10.1172/jci.insight.128561. eCollection 2019 Jun 20. JCI Insight. 2019. PMID: 31217345 Free PMC article.
-
R1 motif is the major actin-binding domain of TRIOBP-4.Biochemistry. 2013 Aug 6;52(31):5256-64. doi: 10.1021/bi400585h. Epub 2013 Jul 22. Biochemistry. 2013. PMID: 23789641
-
ANKRD24 organizes TRIOBP to reinforce stereocilia insertion points.J Cell Biol. 2022 Apr 4;221(4):e202109134. doi: 10.1083/jcb.202109134. Epub 2022 Feb 17. J Cell Biol. 2022. PMID: 35175278 Free PMC article.
-
The actin cytoskeleton in hair bundle development and hearing loss.Hear Res. 2023 Sep 1;436:108817. doi: 10.1016/j.heares.2023.108817. Epub 2023 May 26. Hear Res. 2023. PMID: 37300948 Free PMC article. Review.
-
Stereocilia Rootlets: Actin-Based Structures That Are Essential for Structural Stability of the Hair Bundle.Int J Mol Sci. 2020 Jan 3;21(1):324. doi: 10.3390/ijms21010324. Int J Mol Sci. 2020. PMID: 31947734 Free PMC article. Review.
Cited by
-
A Biophysical Model for the Staircase Geometry of Stereocilia.PLoS One. 2015 Jul 24;10(7):e0127926. doi: 10.1371/journal.pone.0127926. eCollection 2015. PLoS One. 2015. PMID: 26207893 Free PMC article.
-
Neurog1 can partially substitute for Atoh1 function in hair cell differentiation and maintenance during organ of Corti development.Development. 2015 Aug 15;142(16):2810-21. doi: 10.1242/dev.123091. Epub 2015 Jul 24. Development. 2015. PMID: 26209643 Free PMC article.
-
Gene expression analysis of zebrafish melanocytes, iridophores, and retinal pigmented epithelium reveals indicators of biological function and developmental origin.PLoS One. 2013 Jul 9;8(7):e67801. doi: 10.1371/journal.pone.0067801. Print 2013. PLoS One. 2013. PMID: 23874447 Free PMC article.
-
Genetics of pediatric hearing loss: A functional perspective.Laryngoscope Investig Otolaryngol. 2020 May 2;5(3):511-519. doi: 10.1002/lio2.390. eCollection 2020 Jun. Laryngoscope Investig Otolaryngol. 2020. PMID: 32596495 Free PMC article. Review.
-
cAMP and voltage modulate rat auditory mechanotransduction by decreasing the stiffness of gating springs.Proc Natl Acad Sci U S A. 2022 Jul 26;119(30):e2107567119. doi: 10.1073/pnas.2107567119. Epub 2022 Jul 19. Proc Natl Acad Sci U S A. 2022. PMID: 35858439 Free PMC article.
References
-
- Belyantseva IA, Boger ET, Naz S, Frolenkov GI, Sellers JR, Ahmed ZM, Griffith AJ, Friedman TB. Myosin-XVa is required for tip localization of whirlin and differential elongation of hair-cell stereocilia. Nat Cell Biol. 2005;7:148–156. - PubMed
Publication types
MeSH terms
Substances
Associated data
- Actions
- Actions
Grants and funding
- R01 DC008861/DC/NIDCD NIH HHS/United States
- Z01 DK060100/ImNIH/Intramural NIH HHS/United States
- ZIA DC000039-13/ImNIH/Intramural NIH HHS/United States
- Z01 DC000048/ImNIH/Intramural NIH HHS/United States
- R01 DC009434/DC/NIDCD NIH HHS/United States
- WT_/Wellcome Trust/United Kingdom
- 071394/Z/03/Z/WT_/Wellcome Trust/United Kingdom
- R01DC008861/DC/NIDCD NIH HHS/United States
- ZIA DC000048-12/ImNIH/Intramural NIH HHS/United States
- R01DC009434/DC/NIDCD NIH HHS/United States
- Z01 HL004232/ImNIH/Intramural NIH HHS/United States
- Z01 DC000064/ImNIH/Intramural NIH HHS/United States
- R00 DC009287/DC/NIDCD NIH HHS/United States
- R01 DC004314/DC/NIDCD NIH HHS/United States
LinkOut - more resources
Full Text Sources
Other Literature Sources
Medical
Molecular Biology Databases