Cysteine string protein-alpha prevents activity-dependent degeneration in GABAergic synapses
- PMID: 20505105
- PMCID: PMC6632397
- DOI: 10.1523/JNEUROSCI.0924-10.2010
Cysteine string protein-alpha prevents activity-dependent degeneration in GABAergic synapses
Abstract
The continuous release of neurotransmitter could be seen to place a persistent burden on presynaptic proteins, one that could compromise nerve terminal function. This supposition and the molecular mechanisms that might protect highly active synapses merit investigation. In hippocampal cultures from knock-out mice lacking the presynaptic cochaperone cysteine string protein-alpha (CSP-alpha), we observe progressive degeneration of highly active synaptotagmin 2 (Syt2)-expressing GABAergic synapses, but surprisingly not of glutamatergic terminals. In CSP-alpha knock-out mice, synaptic degeneration of basket cell terminals occurs in vivo in the presence of normal glutamatergic synapses onto dentate gyrus granule cells. Consistent with this, in hippocampal cultures from these mice, the frequency of miniature IPSCs, caused by spontaneous GABA release, progressively declines, whereas the frequency of miniature excitatory AMPA receptor-mediated currents (mEPSCs), caused by spontaneous release of glutamate, is normal. However, the mEPSC amplitude progressively decreases. Remarkably, long-term block of glutamatergic transmission in cultures lacking CSP-alpha substantially rescues Syt2-expressing GABAergic synapses from neurodegeneration. These findings demonstrate that elevated neural activity increases synapse vulnerability and that CSP-alpha is essential to maintain presynaptic function under a physiologically high-activity regimen.
Figures
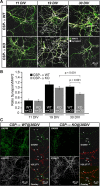
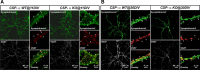
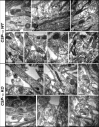
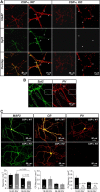
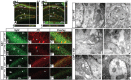
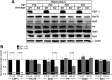
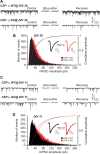
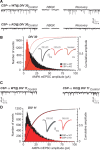
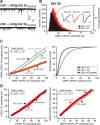
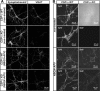
Similar articles
-
Sequential postsynaptic maturation governs the temporal order of GABAergic and glutamatergic synaptogenesis in rat embryonic cultures.J Neurosci. 2007 Oct 3;27(40):10860-9. doi: 10.1523/JNEUROSCI.2744-07.2007. J Neurosci. 2007. PMID: 17913919 Free PMC article.
-
Presynaptically silent GABA synapses in hippocampus.J Neurosci. 2005 Apr 20;25(16):4031-9. doi: 10.1523/JNEUROSCI.4969-04.2005. J Neurosci. 2005. PMID: 15843605 Free PMC article.
-
An Optogenetic Approach for Investigation of Excitatory and Inhibitory Network GABA Actions in Mice Expressing Channelrhodopsin-2 in GABAergic Neurons.J Neurosci. 2016 Jun 1;36(22):5961-73. doi: 10.1523/JNEUROSCI.3482-15.2016. J Neurosci. 2016. PMID: 27251618 Free PMC article.
-
The synaptic split of SNAP-25: different roles in glutamatergic and GABAergic neurons?Neuroscience. 2009 Jan 12;158(1):223-30. doi: 10.1016/j.neuroscience.2008.03.014. Epub 2008 Mar 20. Neuroscience. 2009. PMID: 18514426 Review. No abstract available.
-
Synaptic inhibition and γ-aminobutyric acid in the mammalian central nervous system.Proc Jpn Acad Ser B Phys Biol Sci. 2013;89(4):139-56. doi: 10.2183/pjab.89.139. Proc Jpn Acad Ser B Phys Biol Sci. 2013. PMID: 23574805 Free PMC article. Review.
Cited by
-
Synaptotagmin-2 is a reliable marker for parvalbumin positive inhibitory boutons in the mouse visual cortex.PLoS One. 2012;7(4):e35323. doi: 10.1371/journal.pone.0035323. Epub 2012 Apr 23. PLoS One. 2012. PMID: 22539967 Free PMC article.
-
Structure of the Golgi apparatus is not influenced by a GAG deletion mutation in the dystonia-associated gene Tor1a.PLoS One. 2018 Nov 7;13(11):e0206123. doi: 10.1371/journal.pone.0206123. eCollection 2018. PLoS One. 2018. PMID: 30403723 Free PMC article.
-
Cysteine string protein alpha accumulates with early pre-synaptic dysfunction in Alzheimer's disease.Brain Commun. 2022 Jul 23;4(4):fcac192. doi: 10.1093/braincomms/fcac192. eCollection 2022. Brain Commun. 2022. PMID: 35928052 Free PMC article.
-
The involvement of Purkinje cells in progressive myoclonic epilepsy: Focus on neuronal ceroid lipofuscinosis.Neurobiol Dis. 2023 Sep;185:106258. doi: 10.1016/j.nbd.2023.106258. Epub 2023 Aug 11. Neurobiol Dis. 2023. PMID: 37573956 Free PMC article. Review.
-
Overlapping role of dynamin isoforms in synaptic vesicle endocytosis.Neuron. 2011 Jun 23;70(6):1100-14. doi: 10.1016/j.neuron.2011.04.031. Neuron. 2011. PMID: 21689597 Free PMC article.
References
-
- Chandra S, Gallardo G, Fernández-Chacón R, Schlüter OM, Südhof TC. Alpha-synuclein cooperates with CSPalpha in preventing neurodegeneration. Cell. 2005;123:383–396. - PubMed
-
- Craig AM, Blackstone CD, Huganir RL, Banker G. The distribution of glutamate receptors in cultured rat hippocampal neurons: postsynaptic clustering of AMPA-selective subunits. Neuron. 1993;10:1055–1068. - PubMed
Publication types
MeSH terms
Substances
LinkOut - more resources
Full Text Sources
Molecular Biology Databases