Multiple Myo4 motors enhance ASH1 mRNA transport in Saccharomyces cerevisiae
- PMID: 20457760
- PMCID: PMC2872910
- DOI: 10.1083/jcb.200912011
Multiple Myo4 motors enhance ASH1 mRNA transport in Saccharomyces cerevisiae
Abstract
In Saccharomyces cerevisiae, ASH1 mRNA is transported to the bud tip by the class V myosin Myo4. In vivo, Myo4 moves RNA in a rapid and continuous fashion, but in vitro Myo4 is a nonprocessive, monomeric motor that forms a complex with She3. To understand how nonprocessive motors generate continuous transport, we used a novel purification method to show that Myo4, She3, and the RNA-binding protein She2 are the sole major components of an active ribonucleoprotein transport unit. We demonstrate that a single localization element contains multiple copies of Myo4 and a tetramer of She2, which suggests that She2 may recruit multiple motors to an RNA. Furthermore, we show that increasing the number of Myo4-She3 molecules bound to ASH1 RNA in the absence of She2 increases the efficiency of RNA transport to the bud. Our data suggest that multiple, nonprocessive Myo4 motors can generate continuous transport of mRNA to the bud tip.
Figures
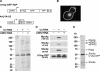
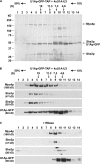
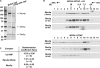
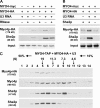
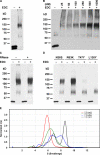
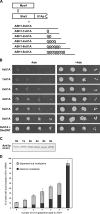
Similar articles
-
ASH1 mRNP-core factors form stable complexes in absence of cargo RNA at physiological conditions.RNA Biol. 2015;12(3):233-7. doi: 10.1080/15476286.2015.1017217. RNA Biol. 2015. PMID: 25826656 Free PMC article.
-
Essential features of the class V myosin from budding yeast for ASH1 mRNA transport.Mol Biol Cell. 2009 Jul;20(14):3414-21. doi: 10.1091/mbc.e08-08-0801. Epub 2009 May 28. Mol Biol Cell. 2009. PMID: 19477930 Free PMC article.
-
She3p possesses a novel activity required for ASH1 mRNA localization in Saccharomyces cerevisiae.Eukaryot Cell. 2009 Jul;8(7):1072-83. doi: 10.1128/EC.00084-09. Epub 2009 May 8. Eukaryot Cell. 2009. PMID: 19429778 Free PMC article.
-
Here, there, everywhere. mRNA localization in budding yeast.RNA Biol. 2014;11(8):1031-9. doi: 10.4161/rna.29945. Epub 2014 Oct 31. RNA Biol. 2014. PMID: 25482891 Free PMC article. Review.
-
RNA localization: SHEdding light on the RNA-motor linkage.Curr Biol. 2001 Mar 6;11(5):R166-8. doi: 10.1016/s0960-9822(01)00084-7. Curr Biol. 2001. PMID: 11267883 Review.
Cited by
-
A numbers game underpins cytoplasmic mRNA transport.Nat Cell Biol. 2012 Apr 2;14(4):333-5. doi: 10.1038/ncb2475. Nat Cell Biol. 2012. PMID: 22469827
-
Aptamers in analytics.Analyst. 2016 Mar 7;141(5):1551-68. doi: 10.1039/c5an01824b. Analyst. 2016. PMID: 26864075 Free PMC article. Review.
-
ASH1 mRNP-core factors form stable complexes in absence of cargo RNA at physiological conditions.RNA Biol. 2015;12(3):233-7. doi: 10.1080/15476286.2015.1017217. RNA Biol. 2015. PMID: 25826656 Free PMC article.
-
Molecular motors: directing traffic during RNA localization.Crit Rev Biochem Mol Biol. 2011 Jun;46(3):229-39. doi: 10.3109/10409238.2011.572861. Epub 2011 Apr 11. Crit Rev Biochem Mol Biol. 2011. PMID: 21476929 Free PMC article. Review.
-
Control of cytoplasmic mRNA localization.Cell Mol Life Sci. 2012 Feb;69(4):535-52. doi: 10.1007/s00018-011-0814-3. Epub 2011 Oct 8. Cell Mol Life Sci. 2012. PMID: 21984598 Free PMC article. Review.
References
Publication types
MeSH terms
Substances
Grants and funding
LinkOut - more resources
Full Text Sources
Molecular Biology Databases