The NADPH oxidase subunit NOX4 is a new target gene of the hypoxia-inducible factor-1
- PMID: 20427574
- PMCID: PMC2883952
- DOI: 10.1091/mbc.e09-12-1003
The NADPH oxidase subunit NOX4 is a new target gene of the hypoxia-inducible factor-1
Abstract
NADPH oxidases are important sources of reactive oxygen species (ROS), possibly contributing to various disorders associated with enhanced proliferation. NOX4 appears to be involved in vascular signaling and may contribute to the response to hypoxia. However, the exact mechanisms controlling NOX4 levels under hypoxia are not resolved. We found that hypoxia rapidly enhanced NOX4 mRNA and protein levels in pulmonary artery smooth-muscle cells (PASMCs) as well as in pulmonary vessels from mice exposed to hypoxia. This response was dependent on the hypoxia-inducible transcription factor HIF-1alpha because overexpression of HIF-1alpha increased NOX4 expression, whereas HIF-1alpha depletion prevented this response. Mutation of a putative hypoxia-responsive element in the NOX4 promoter abolished hypoxic and HIF-1alpha-induced activation of the NOX4 promoter. Chromatin immunoprecipitation confirmed HIF-1alpha binding to the NOX4 gene. Induction of NOX4 by HIF-1alpha contributed to maintain ROS levels after hypoxia and hypoxia-induced proliferation of PASMCs. These findings show that NOX4 is a new target gene of HIF-1alpha involved in the response to hypoxia. Together with our previous findings that NOX4 mediates HIF-1alpha induction under normoxia, these data suggest an important role of the signaling axis between NOX4 and HIF-1alpha in various cardiovascular disorders under hypoxic and also nonhypoxic conditions.
Figures
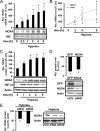
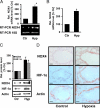
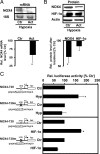
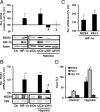
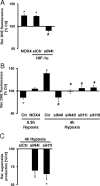
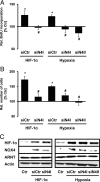
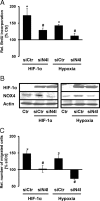
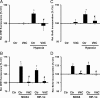
Similar articles
-
NADPH oxidase 4 mediates insulin-stimulated HIF-1α and VEGF expression, and angiogenesis in vitro.PLoS One. 2012;7(10):e48393. doi: 10.1371/journal.pone.0048393. Epub 2012 Oct 29. PLoS One. 2012. PMID: 23144758 Free PMC article.
-
[Resveratrol inhibits hypoxia-induced oxidative stress and proliferation in pulmonary artery smooth muscle cells through the HIF-1α/NOX4/ROS signaling pathway].Sheng Li Xue Bao. 2020 Oct 25;72(5):551-558. Sheng Li Xue Bao. 2020. PMID: 33106825 Chinese.
-
Differential transcriptional regulation of hypoxia-inducible factor-1α by arsenite under normoxia and hypoxia: involvement of Nrf2.J Mol Med (Berl). 2016 Oct;94(10):1153-1166. doi: 10.1007/s00109-016-1439-7. Epub 2016 Jun 10. J Mol Med (Berl). 2016. PMID: 27286880 Free PMC article.
-
Hypoxia and the endometrium: An indispensable role for HIF-1α as therapeutic strategies.Redox Biol. 2024 Jul;73:103205. doi: 10.1016/j.redox.2024.103205. Epub 2024 May 21. Redox Biol. 2024. PMID: 38815332 Free PMC article. Review.
-
Hypoxia and free radicals: role in tumor progression and the use of engineering-based platforms to address these relationships.Free Radic Biol Med. 2015 Feb;79:281-91. doi: 10.1016/j.freeradbiomed.2014.09.015. Epub 2014 Oct 22. Free Radic Biol Med. 2015. PMID: 25257256 Free PMC article. Review.
Cited by
-
Nox4-generated superoxide drives angiotensin II-induced neural stem cell proliferation.Dev Neurosci. 2013;35(4):293-305. doi: 10.1159/000350502. Epub 2013 Jun 8. Dev Neurosci. 2013. PMID: 23751520 Free PMC article.
-
NADPH oxidases in vascular pathology.Antioxid Redox Signal. 2014 Jun 10;20(17):2794-814. doi: 10.1089/ars.2013.5607. Epub 2013 Nov 1. Antioxid Redox Signal. 2014. PMID: 24180474 Free PMC article. Review.
-
H2O2 Metabolism in Normal Thyroid Cells and in Thyroid Tumorigenesis: Focus on NADPH Oxidases.Antioxidants (Basel). 2019 May 10;8(5):126. doi: 10.3390/antiox8050126. Antioxidants (Basel). 2019. PMID: 31083324 Free PMC article. Review.
-
The Warburg Effect in Diabetic Kidney Disease.Semin Nephrol. 2018 Mar;38(2):111-120. doi: 10.1016/j.semnephrol.2018.01.002. Semin Nephrol. 2018. PMID: 29602394 Free PMC article. Review.
-
Metabolic zonation of the liver: The oxygen gradient revisited.Redox Biol. 2017 Apr;11:622-630. doi: 10.1016/j.redox.2017.01.012. Epub 2017 Jan 17. Redox Biol. 2017. PMID: 28126520 Free PMC article. Review.
References
-
- Archer S. L., Gomberg-Maitland M., Maitland M. L., Rich S., Garcia J. G., Weir E. K. Mitochondrial metabolism, redox signaling, and fusion: a mitochondria-ROS-HIF-1alpha-Kv1.5 O2-sensing pathway at the intersection of pulmonary hypertension and cancer. Am. J. Physiol. Heart Circ. Physiol. 2008;294:H570–H578. - PubMed
-
- Babior B. M. NADPH oxidase: an update. Blood. 1999;93:1464–1476. - PubMed
-
- Babior B. M., Lambeth J. D., Nauseef W. The neutrophil NADPH oxidase. Arch. Biochem. Biophys. 2002;397:342–344. - PubMed
-
- Bedard K., Krause K. H. The NOX family of ROS-generating NADPH oxidases: physiology and pathophysiology. Physiol. Rev. 2007;87:245–313. - PubMed
Publication types
MeSH terms
Substances
LinkOut - more resources
Full Text Sources
Other Literature Sources