Rad52 SUMOylation affects the efficiency of the DNA repair
- PMID: 20371517
- PMCID: PMC2919706
- DOI: 10.1093/nar/gkq195
Rad52 SUMOylation affects the efficiency of the DNA repair
Erratum in
- Nucleic Acids Res. 2012 Apr;40(8):3775
-
Correction to 'Rad52 SUMOylation affects the efficiency of the DNA repair'.Nucleic Acids Res. 2023 Feb 28;51(4):1998. doi: 10.1093/nar/gkad065. Nucleic Acids Res. 2023. PMID: 36715321 Free PMC article. No abstract available.
Abstract
Homologous recombination (HR) plays a vital role in DNA metabolic processes including meiosis, DNA repair, DNA replication and rDNA homeostasis. HR defects can lead to pathological outcomes, including genetic diseases and cancer. Recent studies suggest that the post-translational modification by the small ubiquitin-like modifier (SUMO) protein plays an important role in mitotic and meiotic recombination. However, the precise role of SUMOylation during recombination is still unclear. Here, we characterize the effect of SUMOylation on the biochemical properties of the Saccharomyces cerevisiae recombination mediator protein Rad52. Interestingly, Rad52 SUMOylation is enhanced by single-stranded DNA, and we show that SUMOylation of Rad52 also inhibits its DNA binding and annealing activities. The biochemical effects of SUMO modification in vitro are accompanied by a shorter duration of spontaneous Rad52 foci in vivo and a shift in spontaneous mitotic recombination from single-strand annealing to gene conversion events in the SUMO-deficient Rad52 mutants. Taken together, our results highlight the importance of Rad52 SUMOylation as part of a 'quality control' mechanism regulating the efficiency of recombination and DNA repair.
Figures
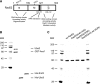
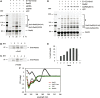
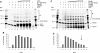
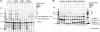
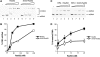
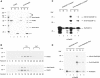
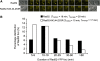
Similar articles
-
SUMOylation of Rad52-Rad59 synergistically change the outcome of mitotic recombination.DNA Repair (Amst). 2016 Jun;42:11-25. doi: 10.1016/j.dnarep.2016.04.001. Epub 2016 Apr 16. DNA Repair (Amst). 2016. PMID: 27130983 Free PMC article.
-
Role of Cdc48/p97 as a SUMO-targeted segregase curbing Rad51-Rad52 interaction.Nat Cell Biol. 2013 May;15(5):526-32. doi: 10.1038/ncb2729. Epub 2013 Apr 28. Nat Cell Biol. 2013. PMID: 23624404
-
Dynamic regulatory interactions of rad51, rad52, and replication protein-a in recombination intermediates.J Mol Biol. 2009 Jul 3;390(1):45-55. doi: 10.1016/j.jmb.2009.05.009. Epub 2009 May 13. J Mol Biol. 2009. PMID: 19445949
-
Regulation of rDNA stability by sumoylation.DNA Repair (Amst). 2009 Apr 5;8(4):507-16. doi: 10.1016/j.dnarep.2009.01.015. Epub 2009 Mar 3. DNA Repair (Amst). 2009. PMID: 19261548 Review.
-
Emerging non-canonical roles for the Rad51-Rad52 interaction in response to double-strand breaks in yeast.Curr Genet. 2020 Oct;66(5):917-926. doi: 10.1007/s00294-020-01081-z. Epub 2020 May 12. Curr Genet. 2020. PMID: 32399607 Free PMC article. Review.
Cited by
-
Caffeine inhibits gene conversion by displacing Rad51 from ssDNA.Nucleic Acids Res. 2015 Aug 18;43(14):6902-18. doi: 10.1093/nar/gkv525. Epub 2015 May 27. Nucleic Acids Res. 2015. PMID: 26019181 Free PMC article.
-
Small ubiquitin-like modifier (SUMO) isoforms and conjugation-independent function in DNA double-strand break repair pathways.J Biol Chem. 2014 Aug 1;289(31):21289-95. doi: 10.1074/jbc.C114.582122. Epub 2014 Jun 25. J Biol Chem. 2014. PMID: 24966330 Free PMC article.
-
Mechanisms and regulation of mitotic recombination in Saccharomyces cerevisiae.Genetics. 2014 Nov;198(3):795-835. doi: 10.1534/genetics.114.166140. Genetics. 2014. PMID: 25381364 Free PMC article. Review.
-
Molecular pathways: understanding the role of Rad52 in homologous recombination for therapeutic advancement.Clin Cancer Res. 2012 Dec 1;18(23):6400-6. doi: 10.1158/1078-0432.CCR-11-3150. Epub 2012 Oct 15. Clin Cancer Res. 2012. PMID: 23071261 Free PMC article. Review.
-
Rad52 SUMOylation functions as a molecular switch that determines a balance between the Rad51- and Rad59-dependent survivors.iScience. 2021 Feb 25;24(3):102231. doi: 10.1016/j.isci.2021.102231. eCollection 2021 Mar 19. iScience. 2021. PMID: 33748714 Free PMC article.
References
-
- Krogh BO, Symington LS. Recombination proteins in yeast. Ann. Rev. Genet. 2004;38:233–271. - PubMed
-
- Sung P, Klein H. Mechanism of homologous recombination: mediators and helicases take on regulatory functions. Nat. Rev. Mol. Cell Biol. 2006;7:739–750. - PubMed
-
- Sung P. Function of yeast Rad52 protein as a mediator between replication protein A and the Rad51 recombinase. J. Biol. Chem. 1997;272:28194–28197. - PubMed
Publication types
MeSH terms
Substances
Grants and funding
LinkOut - more resources
Full Text Sources
Molecular Biology Databases
Research Materials