Prolyl 3-hydroxylase 1 null mice display abnormalities in fibrillar collagen-rich tissues such as tendons, skin, and bones
- PMID: 20363744
- PMCID: PMC2878055
- DOI: 10.1074/jbc.M110.102228
Prolyl 3-hydroxylase 1 null mice display abnormalities in fibrillar collagen-rich tissues such as tendons, skin, and bones
Erratum in
- J Biol Chem. 2010 Jun 25;285(26):20421
Abstract
Osteogenesis imperfecta (OI) is a skeletal disorder primarily caused by mutations in the type I collagen genes. However, recent investigations have revealed that mutations in the genes encoding for cartilage-associated protein (CRTAP) or prolyl 3-hydroxylase 1 (P3H1) can cause a severe, recessive form of OI. These reports show minimal 3-hydroxylation of key proline residues in type I collagen as a result of CRTAP or P3H1 deficiency and demonstrate the importance of P3H1 and CRTAP to bone structure and development. P3H1 and CRTAP have previously been shown to form a stable complex with cyclophilin B, and P3H1 was shown to catalyze the 3-hydroxylation of specific proline residues in procollagen I in vitro. Here we describe a mouse model in which the P3H1 gene has been inactivated. Our data demonstrate abnormalities in collagen fibril ultrastructure in tendons from P3H1 null mice by electron microscopy. Differences are also seen in skin architecture, as well as in developing limbs by histology. Additionally bone mass and strength were significantly lower in the P3H1 mice as compared with wild-type littermates. Altogether these investigations demonstrate disturbances of collagen fiber architecture in tissues rich in fibrillar collagen, including bone, tendon, and skin. This model system presents a good opportunity to study the underlying mechanisms of recessive OI and to better understand its effects in humans.
Figures
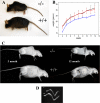
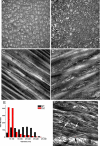
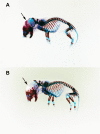
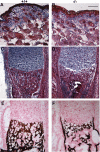
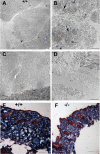
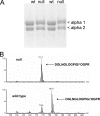
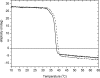
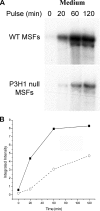
Similar articles
-
Severe osteogenesis imperfecta in cyclophilin B-deficient mice.PLoS Genet. 2009 Dec;5(12):e1000750. doi: 10.1371/journal.pgen.1000750. Epub 2009 Dec 4. PLoS Genet. 2009. PMID: 19997487 Free PMC article.
-
PPIB mutations cause severe osteogenesis imperfecta.Am J Hum Genet. 2009 Oct;85(4):521-7. doi: 10.1016/j.ajhg.2009.09.001. Epub 2009 Sep 24. Am J Hum Genet. 2009. PMID: 19781681 Free PMC article.
-
Prolyl 3-hydroxylase 1 and CRTAP are mutually stabilizing in the endoplasmic reticulum collagen prolyl 3-hydroxylation complex.Hum Mol Genet. 2010 Jan 15;19(2):223-34. doi: 10.1093/hmg/ddp481. Epub 2009 Oct 21. Hum Mol Genet. 2010. PMID: 19846465 Free PMC article.
-
Null mutations in LEPRE1 and CRTAP cause severe recessive osteogenesis imperfecta.Cell Tissue Res. 2010 Jan;339(1):59-70. doi: 10.1007/s00441-009-0872-0. Epub 2009 Oct 28. Cell Tissue Res. 2010. PMID: 19862557 Free PMC article. Review.
-
Components of the collagen prolyl 3-hydroxylation complex are crucial for normal bone development.Cell Cycle. 2007 Jul 15;6(14):1675-81. doi: 10.4161/cc.6.14.4474. Epub 2007 May 18. Cell Cycle. 2007. PMID: 17630507 Review.
Cited by
-
Curative Cell and Gene Therapy for Osteogenesis Imperfecta.J Bone Miner Res. 2022 May;37(5):826-836. doi: 10.1002/jbmr.4549. Epub 2022 Apr 17. J Bone Miner Res. 2022. PMID: 35306687 Free PMC article. Review.
-
Differential effects of collagen prolyl 3-hydroxylation on skeletal tissues.PLoS Genet. 2014 Jan;10(1):e1004121. doi: 10.1371/journal.pgen.1004121. Epub 2014 Jan 23. PLoS Genet. 2014. PMID: 24465224 Free PMC article.
-
Connective tissue alterations in Fkbp10-/- mice.Hum Mol Genet. 2014 Sep 15;23(18):4822-31. doi: 10.1093/hmg/ddu197. Epub 2014 Apr 28. Hum Mol Genet. 2014. PMID: 24777781 Free PMC article.
-
Developmental Stage-dependent Regulation of Prolyl 3-Hydroxylation in Tendon Type I Collagen.J Biol Chem. 2016 Jan 8;291(2):837-47. doi: 10.1074/jbc.M115.686105. Epub 2015 Nov 13. J Biol Chem. 2016. PMID: 26567337 Free PMC article.
-
Aberrant binding of mutant HSP47 affects posttranslational modification of type I collagen and leads to osteogenesis imperfecta.PLoS Genet. 2021 Feb 1;17(2):e1009339. doi: 10.1371/journal.pgen.1009339. eCollection 2021 Feb. PLoS Genet. 2021. PMID: 33524049 Free PMC article.
References
-
- Rauch F., Glorieux F. H. (2004) Lancet 363, 1377–1385 - PubMed
-
- Royce P. M., Steinmann B. (eds) (2002) Connective Tissue and Its Heritable Disorders: Molecular, Genetic, and Medical Aspects, pp. 385–430, Wiley-Liss, New York
-
- Morello R., Bertin T. K., Chen Y., Hicks J., Tonachini L., Monticone M., Castagnola P., Rauch F., Glorieux F. H., Vranka J., Bächinger H. P., Pace J. M., Schwarze U., Byers P. H., Weis M., Fernandes R. J., Eyre D. R., Yao Z., Boyce B. F., Lee B. (2006) Cell 127, 291–304 - PubMed
-
- Baldridge D., Schwarze U., Morello R., Lennington J., Bertin T. K., Pace J. M., Pepin M. G., Weis M., Eyre D. R., Walsh J., Lambert D., Green A., Robinson H., Michelson M., Houge G., Lindman C., Martin J., Ward J., Lemyre E., Mitchell J. J., Krakow D., Rimoin D. L., Cohn D. H., Byers P. H., Lee B. (2008) Hum. Mutat. 29, 1435–1442 - PMC - PubMed
Publication types
MeSH terms
Substances
Grants and funding
LinkOut - more resources
Full Text Sources
Molecular Biology Databases