Regulation of macroautophagy in Saccharomyces cerevisiae
- PMID: 20359542
- PMCID: PMC2930024
- DOI: 10.1016/j.semcdb.2010.03.009
Regulation of macroautophagy in Saccharomyces cerevisiae
Abstract
Macroautophagy (hereafter autophagy) is a cellular degradation process, which in yeast is induced in response to nutrient deprivation. In this process, a double-membrane vesicle, an autophagosome, surrounds part of the cytoplasm and fuses with the vacuole to allow the breakdown and subsequent recycling of the cargo. In yeast, many autophagy-related (ATG) genes have been identified that are required for selective and/or nonselective autophagy. In all autophagy-related pathways, core Atg proteins are required for the formation of the autophagosome, which is one of the most unique aspects of autophagy and is unlike other vesicle transport events. In contrast to nonselective autophagy, the selective processes are induced in response to various specific physiological conditions such as alterations in the carbon source. In this review, we provide an overview of the common aspects concerning the mechanism of autophagy-related pathways, and highlight recent advances in our understanding of the machinery that controls autophagy induction in response to nutrient starvation conditions.
(c) 2010 Elsevier Ltd. All rights reserved.
Figures
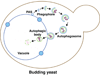
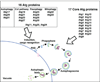
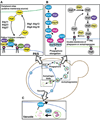
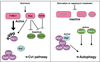
Similar articles
-
Atg41/Icy2 regulates autophagosome formation.Autophagy. 2015;11(12):2288-99. doi: 10.1080/15548627.2015.1107692. Autophagy. 2015. PMID: 26565778 Free PMC article.
-
An overview of macroautophagy in yeast.J Mol Biol. 2016 May 8;428(9 Pt A):1681-99. doi: 10.1016/j.jmb.2016.02.021. Epub 2016 Feb 22. J Mol Biol. 2016. PMID: 26908221 Free PMC article. Review.
-
Molecular machinery of autophagosome formation in yeast, Saccharomyces cerevisiae.FEBS Lett. 2007 May 22;581(11):2156-61. doi: 10.1016/j.febslet.2007.01.096. Epub 2007 Mar 12. FEBS Lett. 2007. PMID: 17382324
-
Mechanistic Insights into the Role of Atg11 in Selective Autophagy.J Mol Biol. 2020 Jan 3;432(1):104-122. doi: 10.1016/j.jmb.2019.06.017. Epub 2019 Jun 22. J Mol Biol. 2020. PMID: 31238043 Free PMC article. Review.
-
Fine mapping of autophagy-related proteins during autophagosome formation in Saccharomyces cerevisiae.J Cell Sci. 2013 Jun 1;126(Pt 11):2534-44. doi: 10.1242/jcs.122960. Epub 2013 Apr 2. J Cell Sci. 2013. PMID: 23549786
Cited by
-
Class IA PI3K p110β subunit promotes autophagy through Rab5 small GTPase in response to growth factor limitation.Mol Cell. 2013 Apr 11;50(1):29-42. doi: 10.1016/j.molcel.2013.01.022. Epub 2013 Feb 21. Mol Cell. 2013. PMID: 23434372 Free PMC article.
-
Autophagy contributes to regulation of nuclear dynamics during vegetative growth and hyphal fusion in Fusarium oxysporum.Autophagy. 2015;11(1):131-44. doi: 10.4161/15548627.2014.994413. Autophagy. 2015. PMID: 25560310 Free PMC article.
-
The protein factor-arrest 11 (Far11) is essential for the toxicity of human caspase-10 in yeast and participates in the regulation of autophagy and the DNA damage signaling.J Biol Chem. 2012 Aug 24;287(35):29636-47. doi: 10.1074/jbc.M112.344192. Epub 2012 Jul 10. J Biol Chem. 2012. PMID: 22782902 Free PMC article.
-
Histone Acetyltransferase CfGcn5-Mediated Autophagy Governs the Pathogenicity of Colletotrichum fructicola.mBio. 2022 Oct 26;13(5):e0195622. doi: 10.1128/mbio.01956-22. Epub 2022 Aug 17. mBio. 2022. PMID: 35975920 Free PMC article.
-
Hyphal Fusions Enable Efficient Nutrient Distribution in Colletotrichum graminicola Conidiation and Symptom Development on Maize.Microorganisms. 2022 Jun 1;10(6):1146. doi: 10.3390/microorganisms10061146. Microorganisms. 2022. PMID: 35744664 Free PMC article.
References
Publication types
MeSH terms
Substances
Grants and funding
LinkOut - more resources
Full Text Sources
Other Literature Sources
Molecular Biology Databases