Differential effects of sumoylation on transcription and alternative splicing by transcription elongation regulator 1 (TCERG1)
- PMID: 20215116
- PMCID: PMC2865347
- DOI: 10.1074/jbc.M109.063750
Differential effects of sumoylation on transcription and alternative splicing by transcription elongation regulator 1 (TCERG1)
Abstract
Modification of proteins by small ubiquitin-like modifier (SUMO) is emerging as an important control of transcription and RNA processing. The human factor TCERG1 (also known as CA150) participates in transcriptional elongation and alternative splicing of pre-mRNAs. Here, we report that SUMO family proteins modify TCERG1. Furthermore, TCERG1 binds to the E2 SUMO-conjugating enzyme Ubc9. Two lysines (Lys-503 and Lys-608) of TCERG1 are the major sumoylation sites. Sumoylation does not affect localization of TCERG1 to the splicing factor-rich nuclear speckles or the alternative splicing function of TCERG1. However, mutation of the SUMO acceptor lysine residues enhanced TCERG1 transcriptional activity, indicating that SUMO modification negatively regulates TCERG1 transcriptional activity. These results reveal a regulatory role for sumoylation in controlling the activity of a transcription factor that modulates RNA polymerase II elongation and mRNA alternative processing, which are discriminated differently by this post-translational modification.
Figures
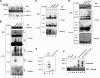
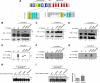
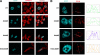
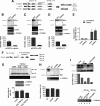
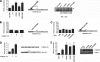
Similar articles
-
TCERG1 regulates alternative splicing of the Bcl-x gene by modulating the rate of RNA polymerase II transcription.Mol Cell Biol. 2012 Feb;32(4):751-62. doi: 10.1128/MCB.06255-11. Epub 2011 Dec 12. Mol Cell Biol. 2012. PMID: 22158966 Free PMC article.
-
Identification of the cellular targets of the transcription factor TCERG1 reveals a prevalent role in mRNA processing.J Biol Chem. 2008 Mar 21;283(12):7949-61. doi: 10.1074/jbc.M709402200. Epub 2008 Jan 10. J Biol Chem. 2008. PMID: 18187414
-
Sumoylation regulates multiple aspects of mammalian poly(A) polymerase function.Genes Dev. 2008 Feb 15;22(4):499-511. doi: 10.1101/gad.1628208. Genes Dev. 2008. PMID: 18281463 Free PMC article.
-
SUMO: getting it on.Biochem Soc Trans. 2007 Dec;35(Pt 6):1409-13. doi: 10.1042/BST0351409. Biochem Soc Trans. 2007. PMID: 18031233 Review.
-
Roles of Sumoylation in mRNA Processing and Metabolism.Adv Exp Med Biol. 2017;963:15-33. doi: 10.1007/978-3-319-50044-7_2. Adv Exp Med Biol. 2017. PMID: 28197904 Free PMC article. Review.
Cited by
-
Evolution of the Early Spliceosomal Complex-From Constitutive to Regulated Splicing.Int J Mol Sci. 2021 Nov 18;22(22):12444. doi: 10.3390/ijms222212444. Int J Mol Sci. 2021. PMID: 34830325 Free PMC article. Review.
-
The role of MeCP2 in learning and memory.Learn Mem. 2019 Aug 15;26(9):343-350. doi: 10.1101/lm.048876.118. Print 2019 Sep. Learn Mem. 2019. PMID: 31416907 Free PMC article.
-
Intramolecular autoinhibition regulates the selectivity of PRPF40A tandem WW domains for proline-rich motifs.Nat Commun. 2024 May 8;15(1):3888. doi: 10.1038/s41467-024-48004-x. Nat Commun. 2024. PMID: 38719828 Free PMC article.
-
Roles for SUMO in pre-mRNA processing.Wiley Interdiscip Rev RNA. 2016 Jan-Feb;7(1):105-12. doi: 10.1002/wrna.1318. Epub 2015 Nov 13. Wiley Interdiscip Rev RNA. 2016. PMID: 26563097 Free PMC article. Review.
-
The in vivo dynamics of TCERG1, a factor that couples transcriptional elongation with splicing.RNA. 2016 Apr;22(4):571-82. doi: 10.1261/rna.052795.115. Epub 2016 Feb 12. RNA. 2016. PMID: 26873599 Free PMC article.
References
-
- Proudfoot N. J., Furger A., Dye M. J. (2002) Cell 108, 501–512 - PubMed
-
- Auboeuf D., Hönig A., Berget S. M., O'Malley B. W. (2002) Science 298, 416–419 - PubMed
-
- Nogues G., Kadener S., Cramer P., Bentley D., Kornblihtt A. R. (2002) J. Biol. Chem. 277, 43110–43114 - PubMed
-
- Kornblihtt A. R. (2005) Curr. Opin. Cell Biol. 17, 262–268 - PubMed
Publication types
MeSH terms
Substances
LinkOut - more resources
Full Text Sources
Molecular Biology Databases
Miscellaneous