Deposition and biokinetics of inhaled nanoparticles
- PMID: 20205860
- PMCID: PMC2826283
- DOI: 10.1186/1743-8977-7-2
Deposition and biokinetics of inhaled nanoparticles
Abstract
Particle biokinetics is important in hazard identification and characterization of inhaled particles. Such studies intend to convert external to internal exposure or biologically effective dose, and may help to set limits in that way. Here we focus on the biokinetics of inhaled nanometer sized particles in comparison to micrometer sized ones.The presented approach ranges from inhaled particle deposition probability and retention in the respiratory tract to biokinetics and clearance of particles out of the respiratory tract. Particle transport into the blood circulation (translocation), towards secondary target organs and tissues (accumulation), and out of the body (clearance) is considered. The macroscopically assessed amount of particles in the respiratory tract and secondary target organs provides dose estimates for toxicological studies on the level of the whole organism. Complementary, microscopic analyses at the individual particle level provide detailed information about which cells and subcellular components are the target of inhaled particles. These studies contribute to shed light on mechanisms and modes of action eventually leading to adverse health effects by inhaled nanoparticles.We review current methods for macroscopic and microscopic analyses of particle deposition, retention and clearance. Existing macroscopic knowledge on particle biokinetics and microscopic views on particle organ interactions are discussed comparing nanometer and micrometer sized particles. We emphasize the importance for quantitative analyses and the use of particle doses derived from real world exposures.
Figures
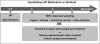
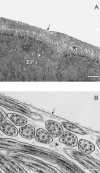
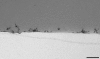
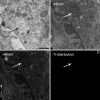
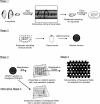
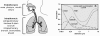
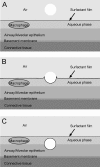
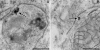
Similar articles
-
Differences in the biokinetics of inhaled nano- versus micrometer-sized particles.Acc Chem Res. 2013 Mar 19;46(3):714-22. doi: 10.1021/ar300043r. Epub 2012 Sep 17. Acc Chem Res. 2013. PMID: 22980029 Free PMC article.
-
Quantitative biokinetics over a 28 day period of freshly generated, pristine, 20 nm titanium dioxide nanoparticle aerosols in healthy adult rats after a single two-hour inhalation exposure.Part Fibre Toxicol. 2019 Jul 9;16(1):29. doi: 10.1186/s12989-019-0303-7. Part Fibre Toxicol. 2019. PMID: 31288843 Free PMC article.
-
Deposition efficiency of inhaled particles (15-5000 nm) related to breathing pattern and lung function: an experimental study in healthy children and adults.Part Fibre Toxicol. 2017 Apr 8;14(1):10. doi: 10.1186/s12989-017-0190-8. Part Fibre Toxicol. 2017. PMID: 28388961 Free PMC article.
-
Significance of particle parameters in the evaluation of exposure-dose-response relationships of inhaled particles.Inhal Toxicol. 1996;8 Suppl:73-89. Inhal Toxicol. 1996. PMID: 11542496 Review.
-
Ultrafine particle-lung interactions: does size matter?J Aerosol Med. 2006 Spring;19(1):74-83. doi: 10.1089/jam.2006.19.74. J Aerosol Med. 2006. PMID: 16551218 Review.
Cited by
-
Differences in the biokinetics of inhaled nano- versus micrometer-sized particles.Acc Chem Res. 2013 Mar 19;46(3):714-22. doi: 10.1021/ar300043r. Epub 2012 Sep 17. Acc Chem Res. 2013. PMID: 22980029 Free PMC article.
-
Titanium dioxide nanomaterials cause endothelial cell leakiness by disrupting the homophilic interaction of VE-cadherin.Nat Commun. 2013;4:1673. doi: 10.1038/ncomms2655. Nat Commun. 2013. PMID: 23575677
-
An in vitro liver model--assessing oxidative stress and genotoxicity following exposure of hepatocytes to a panel of engineered nanomaterials.Part Fibre Toxicol. 2012 Jul 19;9:28. doi: 10.1186/1743-8977-9-28. Part Fibre Toxicol. 2012. PMID: 22812506 Free PMC article.
-
Improving Equitability and Inclusion for Testing and Detection of Lead Poisoning in US Children.Milbank Q. 2023 Mar;101(1):48-73. doi: 10.1111/1468-0009.12596. Epub 2023 Jan 30. Milbank Q. 2023. PMID: 36717973 Free PMC article.
-
Physicochemical characterization of nebulized superparamagnetic iron oxide nanoparticles (SPIONs).J Aerosol Med Pulm Drug Deliv. 2015 Feb;28(1):43-51. doi: 10.1089/jamp.2013.1117. Epub 2014 May 6. J Aerosol Med Pulm Drug Deliv. 2015. PMID: 24801912 Free PMC article.
References
Publication types
MeSH terms
Substances
Grants and funding
LinkOut - more resources
Full Text Sources
Other Literature Sources