Anchorage of VEGF to the extracellular matrix conveys differential signaling responses to endothelial cells
- PMID: 20176926
- PMCID: PMC2828913
- DOI: 10.1083/jcb.200906044
Anchorage of VEGF to the extracellular matrix conveys differential signaling responses to endothelial cells
Abstract
VEGF can be secreted in multiple isoforms with variable affinity for extracellular proteins and different abilities to induce vascular morphogenesis, but the molecular mechanisms behind these effects remain unclear. Here, we show molecular distinctions between signaling initiated from soluble versus matrix-bound VEGF, which mediates a sustained level of VEGFR2 internalization and clustering. Exposure of endothelial cells to matrix-bound VEGF elicits prolonged activation of VEGFR2 with differential phosphorylation of Y1214, and extended activation kinetics of p38. These events require association of VEGFR2 with beta1 integrins. Matrix-bound VEGF also promotes reciprocal responses on beta1 integrin by inducing its association with focal adhesions; a response that is absent upon exposure to soluble VEGF. Inactivation of beta1 integrin blocks the prolonged phosphorylation of Y1214 and consequent activation of p38. Combined, these results indicate that when in the context of extracellular matrix, activation of VEGFR2 is distinct from that of soluble VEGF in terms of recruitment of receptor partners, phosphorylation kinetics, and activation of downstream effectors.
Figures
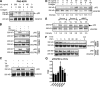
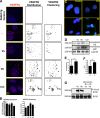
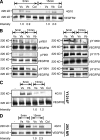
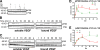
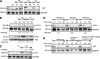
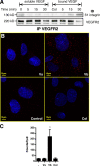
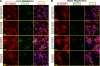
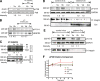
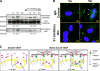
Similar articles
-
Phosphorylation of tyrosine 1214 on VEGFR2 is required for VEGF-induced activation of Cdc42 upstream of SAPK2/p38.Oncogene. 2004 Jan 15;23(2):434-45. doi: 10.1038/sj.onc.1207034. Oncogene. 2004. PMID: 14724572
-
Integrin alphavbeta3, requirement for VEGFR2-mediated activation of SAPK2/p38 and for Hsp90-dependent phosphorylation of focal adhesion kinase in endothelial cells activated by VEGF.Cell Stress Chaperones. 2003 Spring;8(1):37-52. doi: 10.1379/1466-1268(2003)8<37:ivrfva>2.0.co;2. Cell Stress Chaperones. 2003. PMID: 12820653 Free PMC article.
-
Tetraspanin CD63 promotes vascular endothelial growth factor receptor 2-β1 integrin complex formation, thereby regulating activation and downstream signaling in endothelial cells in vitro and in vivo.J Biol Chem. 2013 Jun 28;288(26):19060-71. doi: 10.1074/jbc.M113.468199. Epub 2013 Apr 30. J Biol Chem. 2013. PMID: 23632027 Free PMC article.
-
Molecular Pharmacology of VEGF-A Isoforms: Binding and Signalling at VEGFR2.Int J Mol Sci. 2018 Apr 23;19(4):1264. doi: 10.3390/ijms19041264. Int J Mol Sci. 2018. PMID: 29690653 Free PMC article. Review.
-
VEGF-A-stimulated signalling in endothelial cells via a dual receptor tyrosine kinase system is dependent on co-ordinated trafficking and proteolysis.Biochem Soc Trans. 2009 Dec;37(Pt 6):1193-7. doi: 10.1042/BST0371193. Biochem Soc Trans. 2009. PMID: 19909245 Review.
Cited by
-
Flt-1 (vascular endothelial growth factor receptor-1) is essential for the vascular endothelial growth factor-Notch feedback loop during angiogenesis.Arterioscler Thromb Vasc Biol. 2013 Aug;33(8):1952-9. doi: 10.1161/ATVBAHA.113.301805. Epub 2013 Jun 6. Arterioscler Thromb Vasc Biol. 2013. PMID: 23744993 Free PMC article.
-
Colloidal Gels for Guiding Endothelial Cell Organization via Microstructural Morphology.ACS Appl Mater Interfaces. 2019 Sep 4;11(35):31709-31728. doi: 10.1021/acsami.9b11293. Epub 2019 Aug 21. ACS Appl Mater Interfaces. 2019. PMID: 31403768 Free PMC article.
-
Mechanoregulation of Vascular Endothelial Growth Factor Receptor 2 in Angiogenesis.Front Cardiovasc Med. 2022 Jan 11;8:804934. doi: 10.3389/fcvm.2021.804934. eCollection 2021. Front Cardiovasc Med. 2022. PMID: 35087885 Free PMC article. Review.
-
Spatiotemporally controlled, aptamers-mediated growth factor release locally manipulates microvasculature formation within engineered tissues.Bioact Mater. 2021 Oct 23;12:71-84. doi: 10.1016/j.bioactmat.2021.10.024. eCollection 2022 Jun. Bioact Mater. 2021. PMID: 35087964 Free PMC article.
-
Evolution of the VEGF-regulated vascular network from a neural guidance system.Mol Neurobiol. 2011 Jun;43(3):192-206. doi: 10.1007/s12035-011-8167-3. Epub 2011 Jan 28. Mol Neurobiol. 2011. PMID: 21271303
References
-
- Anderson S.M., Chen T.T., Iruela-Arispe M.L., Segura T. 2009. The phosphorylation of vascular endothelial growth factor receptor-2 (VEGFR-2) by engineered surfaces with electrostatically or covalently immobilized VEGF. Biomaterials. 30:4618–4628 10.1016/j.biomaterials.2009.05.030 - DOI - PMC - PubMed
-
- Ashikari-Hada S., Habuchi H., Kariya Y., Kimata K. 2005. Heparin regulates vascular endothelial growth factor165-dependent mitogenic activity, tube formation, and its receptor phosphorylation of human endothelial cells. Comparison of the effects of heparin and modified heparins. J. Biol. Chem. 280:31508–31515 10.1074/jbc.M414581200 - DOI - PubMed
-
- Bates D.O., Cui T.G., Doughty J.M., Winkler M., Sugiono M., Shields J.D., Peat D., Gillatt D., Harper S.J. 2002. VEGF165b, an inhibitory splice variant of vascular endothelial growth factor, is down-regulated in renal cell carcinoma. Cancer Res. 62:4123–4131 - PubMed
Publication types
MeSH terms
Substances
Grants and funding
LinkOut - more resources
Full Text Sources
Other Literature Sources
Molecular Biology Databases