Metabolic control of G1-S transition: cyclin E degradation by p53-induced activation of the ubiquitin-proteasome system
- PMID: 20176921
- PMCID: PMC2828916
- DOI: 10.1083/jcb.200912024
Metabolic control of G1-S transition: cyclin E degradation by p53-induced activation of the ubiquitin-proteasome system
Abstract
Cell cycle progression is precisely regulated by diverse extrinsic and intrinsic cellular factors. Previous genetic analysis in Drosophila melanogaster has shown that disruption of the mitochondrial electron transport chain activates a G1-S checkpoint as a result of a control of cyclin E by p53. This regulation does not involve activation of the p27 homologue dacapo in flies. We demonstrate that regulation of cyclin E is not at the level of transcription or translation. Rather, attenuated mitochondrial activity leads to transcriptional upregulation of the F-box protein archipelago, the Fbxw7 homologue in flies. We establish that archipelago and the proteasomal machinery contribute to degradation of cyclin E in response to mitochondrial dysfunction. Our work provides in vivo genetic evidence for p53-mediated integration of metabolic stress signals, which modulate the activity of the ubiquitin-proteasome system to degrade cyclin E protein and thereby impose cell cycle arrest.
Figures
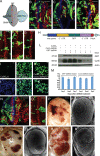
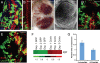
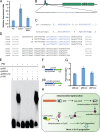
Similar articles
-
dp53 Restrains ectopic neural stem cell formation in the Drosophila brain in a non-apoptotic mechanism involving Archipelago and cyclin E.PLoS One. 2011;6(11):e28098. doi: 10.1371/journal.pone.0028098. Epub 2011 Nov 28. PLoS One. 2011. PMID: 22140513 Free PMC article.
-
Mitochondrial regulation of cell cycle progression during development as revealed by the tenured mutation in Drosophila.Dev Cell. 2005 Dec;9(6):843-54. doi: 10.1016/j.devcel.2005.11.006. Dev Cell. 2005. PMID: 16326395
-
Control of cell cycle entry and exiting from the second mitotic wave in the Drosophila developing eye.BMC Dev Biol. 2008 Jan 24;8:7. doi: 10.1186/1471-213X-8-7. BMC Dev Biol. 2008. PMID: 18218125 Free PMC article.
-
Regulation of the cell cycle at the G1-S transition by proteolysis of cyclin E and p27Kip1.Biochem Biophys Res Commun. 2001 Apr 13;282(4):853-60. doi: 10.1006/bbrc.2001.4627. Biochem Biophys Res Commun. 2001. PMID: 11352628 Review.
-
Targeting the ubiquitin-proteasome system to activate wild-type p53 for cancer therapy.Semin Cancer Biol. 2010 Feb;20(1):29-39. doi: 10.1016/j.semcancer.2009.10.004. Epub 2009 Nov 6. Semin Cancer Biol. 2010. PMID: 19897040 Review.
Cited by
-
Metabolic regulation of the cell cycle.Curr Opin Cell Biol. 2013 Dec;25(6):724-9. doi: 10.1016/j.ceb.2013.07.002. Epub 2013 Jul 24. Curr Opin Cell Biol. 2013. PMID: 23890700 Free PMC article. Review.
-
Notch-dependent expression of the archipelago ubiquitin ligase subunit in the Drosophila eye.Development. 2011 Jan;138(2):251-60. doi: 10.1242/dev.054429. Epub 2010 Dec 9. Development. 2011. PMID: 21148181 Free PMC article.
-
GSK3β-dependent cyclin D1 and cyclin E1 degradation is indispensable for NVP-BEZ235 induced G0/G1 arrest in neuroblastoma cells.Cell Cycle. 2017;16(24):2386-2395. doi: 10.1080/15384101.2017.1383577. Epub 2017 Nov 14. Cell Cycle. 2017. PMID: 28980866 Free PMC article.
-
dp53 Restrains ectopic neural stem cell formation in the Drosophila brain in a non-apoptotic mechanism involving Archipelago and cyclin E.PLoS One. 2011;6(11):e28098. doi: 10.1371/journal.pone.0028098. Epub 2011 Nov 28. PLoS One. 2011. PMID: 22140513 Free PMC article.
-
Heterogeneity in mitochondrial morphology and membrane potential is independent of the nuclear division cycle in multinucleate fungal cells.Eukaryot Cell. 2012 Mar;11(3):353-67. doi: 10.1128/EC.05257-11. Epub 2012 Jan 20. Eukaryot Cell. 2012. PMID: 22267774 Free PMC article.
References
Publication types
MeSH terms
Substances
Grants and funding
LinkOut - more resources
Full Text Sources
Molecular Biology Databases
Research Materials
Miscellaneous