The developmental stage of dentate granule cells dictates their contribution to seizure-induced plasticity
- PMID: 20147533
- PMCID: PMC6634015
- DOI: 10.1523/JNEUROSCI.5655-09.2010
The developmental stage of dentate granule cells dictates their contribution to seizure-induced plasticity
Abstract
Dentate granule cell (DGC) neurogenesis persists throughout life in the hippocampal dentate gyrus. In rodent temporal lobe epilepsy models, status epilepticus (SE) stimulates neurogenesis, but many newborn DGCs integrate aberrantly and are hyperexcitable, whereas others may integrate normally and restore inhibition. The overall influence of altered neurogenesis on epileptogenesis is therefore unclear. To better understand the role DGC neurogenesis plays in seizure-induced plasticity, we injected retroviral (RV) reporters to label dividing DGC progenitors at specific times before or after SE, or used x-irradiation to suppress neurogenesis. RV injections 7 weeks before SE to mark DGCs that had matured by the time of SE labeled cells with normal placement and morphology 4 weeks after SE. RV injections 2 or 4 weeks before seizure induction to label cells still developing during SE revealed normally located DGCs exhibiting hilar basal dendrites and mossy fiber sprouting (MFS) when observed 4 weeks after SE. Cells labeled by injecting RV after SE displayed hilar basal dendrites and ectopic migration, but not sprouting, at 28 d after SE; when examined 10 weeks after SE, however, these cells showed robust MFS. Eliminating cohorts of newborn DGCs by focal brain irradiation at specific times before or after SE decreased MFS or hilar ectopic DGCs, supporting the RV labeling results. These findings indicate that developing DGCs exhibit maturation-dependent vulnerability to SE, indicating that abnormal DGC plasticity derives exclusively from aberrantly developing DGCs. Treatments that restore normal DGC development after epileptogenic insults may therefore ameliorate epileptogenic network dysfunction and associated morbidities.
Figures
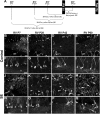
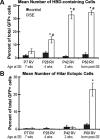
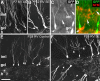
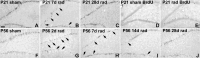
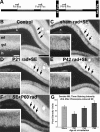
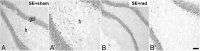
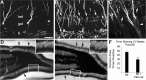
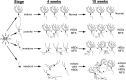
Similar articles
-
Axonal plasticity of age-defined dentate granule cells in a rat model of mesial temporal lobe epilepsy.Neurobiol Dis. 2016 Feb;86:187-96. doi: 10.1016/j.nbd.2015.11.024. Epub 2015 Nov 28. Neurobiol Dis. 2016. PMID: 26644085 Free PMC article.
-
Altered Synaptic Drive onto Birthdated Dentate Granule Cells in Experimental Temporal Lobe Epilepsy.J Neurosci. 2019 Sep 18;39(38):7604-7614. doi: 10.1523/JNEUROSCI.0654-18.2019. Epub 2019 Jul 3. J Neurosci. 2019. PMID: 31270158 Free PMC article.
-
Granule-like neurons at the hilar/CA3 border after status epilepticus and their synchrony with area CA3 pyramidal cells: functional implications of seizure-induced neurogenesis.J Neurosci. 2000 Aug 15;20(16):6144-58. doi: 10.1523/JNEUROSCI.20-16-06144.2000. J Neurosci. 2000. PMID: 10934264 Free PMC article.
-
Chronic epileptogenic cellular alterations in the limbic system after status epilepticus.Epilepsia. 1999;40 Suppl 1:S23-33; discussion S40-1. doi: 10.1111/j.1528-1157.1999.tb00875.x. Epilepsia. 1999. PMID: 10421558 Review.
-
Neurogenesis and Epilepsy.In: Noebels JL, Avoli M, Rogawski MA, Olsen RW, Delgado-Escueta AV, editors. Jasper's Basic Mechanisms of the Epilepsies [Internet]. 4th edition. Bethesda (MD): National Center for Biotechnology Information (US); 2012. In: Noebels JL, Avoli M, Rogawski MA, Olsen RW, Delgado-Escueta AV, editors. Jasper's Basic Mechanisms of the Epilepsies [Internet]. 4th edition. Bethesda (MD): National Center for Biotechnology Information (US); 2012. PMID: 22787618 Free Books & Documents. Review.
Cited by
-
The influence of ectopic migration of granule cells into the hilus on dentate gyrus-CA3 function.PLoS One. 2013 Jun 28;8(6):e68208. doi: 10.1371/journal.pone.0068208. Print 2013. PLoS One. 2013. PMID: 23840835 Free PMC article.
-
Cell-autonomous inactivation of the reelin pathway impairs adult neurogenesis in the hippocampus.J Neurosci. 2012 Aug 29;32(35):12051-65. doi: 10.1523/JNEUROSCI.1857-12.2012. J Neurosci. 2012. PMID: 22933789 Free PMC article.
-
Neuronal network remodeling and Wnt pathway dysregulation in the intra-hippocampal kainate mouse model of temporal lobe epilepsy.PLoS One. 2019 Oct 9;14(10):e0215789. doi: 10.1371/journal.pone.0215789. eCollection 2019. PLoS One. 2019. PMID: 31596871 Free PMC article.
-
VEGF Modulates Neurogenesis and Microvascular Remodeling in Epileptogenesis After Status Epilepticus in Immature Rats.Front Neurol. 2021 Dec 24;12:808568. doi: 10.3389/fneur.2021.808568. eCollection 2021. Front Neurol. 2021. PMID: 35002944 Free PMC article.
-
Depression, stress, epilepsy and adult neurogenesis.Exp Neurol. 2012 Jan;233(1):22-32. doi: 10.1016/j.expneurol.2011.05.023. Epub 2011 Jun 12. Exp Neurol. 2012. PMID: 21684275 Free PMC article. Review.
References
-
- Buckmaster PS, Dudek FE. Neuron loss, granule cell axon reorganization, and functional changes in the dentate gyrus of epileptic kainate-treated rats. J Comp Neurol. 1997;385:385–404. - PubMed
-
- Buckmaster PS, Dudek FE. In vivo intracellular analysis of granule cell axon reorganization in epileptic rats. J Neurosci. 1999;81:712–721. - PubMed
-
- Dashtipour K, Tran PH, Okazaki MM, Nadler JV, Ribak CE. Ultrastructural features and synaptic connections of hilar ectopic granule cells in the rat dentate gyrus are different from those of granule cells in the granule cell layer. Brain Res. 2001;890:261–271. - PubMed
-
- Dashtipour K, Wong AM, Obenaus A, Spigelman I, Ribak CE. Temporal profile of hilar basal dendrite formation on dentate granule cells after status epilepticus. Epilepsy Res. 2003;54:141–151. - PubMed
Publication types
MeSH terms
Grants and funding
LinkOut - more resources
Full Text Sources
Medical