Small-molecule screen identifies reactive oxygen species as key regulators of neutrophil chemotaxis
- PMID: 20142487
- PMCID: PMC2840460
- DOI: 10.1073/pnas.0914351107
Small-molecule screen identifies reactive oxygen species as key regulators of neutrophil chemotaxis
Abstract
Neutrophil chemotaxis plays an essential role in innate immunity, but the underlying cellular mechanism is still not fully characterized. Here, using a small-molecule functional screening, we identified NADPH oxidase-dependent reactive oxygen species as key regulators of neutrophil chemotactic migration. Neutrophils with pharmacologically inhibited oxidase, or isolated from chronic granulomatous disease (CGD) patients and mice, formed more frequent multiple pseudopodia and lost their directionality as they migrated up a chemoattractant concentration gradient. Knocking down NADPH oxidase in differentiated neutrophil-like HL60 cells also led to defective chemotaxis. Consistent with the in vitro results, adoptively transferred CGD murine neutrophils showed impaired in vivo recruitment to sites of inflammation. Together, these results present a physiological role for reactive oxygen species in regulating neutrophil functions and shed light on the pathogenesis of CGD.
Conflict of interest statement
The authors declare no conflict of interest.
Figures
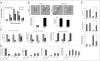
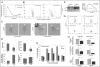
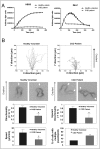
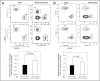
Similar articles
-
Inhibition of human neutrophil IL-8 production by hydrogen peroxide and dysregulation in chronic granulomatous disease.J Immunol. 2005 Jan 1;174(1):411-7. doi: 10.4049/jimmunol.174.1.411. J Immunol. 2005. PMID: 15611265
-
The roles of NADPH oxidase in modulating neutrophil effector responses.Mol Oral Microbiol. 2019 Apr;34(2):27-38. doi: 10.1111/omi.12252. Epub 2019 Feb 7. Mol Oral Microbiol. 2019. PMID: 30632295 Free PMC article. Review.
-
Reactive oxygen species as signaling molecules in neutrophil chemotaxis.Commun Integr Biol. 2010 May;3(3):278-81. doi: 10.4161/cib.3.3.11559. Commun Integr Biol. 2010. PMID: 20714413 Free PMC article.
-
A nox2/cybb zebrafish mutant with defective myeloid cell reactive oxygen species production displays normal initial neutrophil recruitment to sterile tail injuries.G3 (Bethesda). 2024 Jun 5;14(6):jkae079. doi: 10.1093/g3journal/jkae079. G3 (Bethesda). 2024. PMID: 38696730 Free PMC article.
-
Intersecting Stories of the Phagocyte NADPH Oxidase and Chronic Granulomatous Disease.Methods Mol Biol. 2019;1982:3-16. doi: 10.1007/978-1-4939-9424-3_1. Methods Mol Biol. 2019. PMID: 31172463 Review.
Cited by
-
Update of cellular responses to the efferocytosis of necroptosis and pyroptosis.Cell Div. 2023 Apr 9;18(1):5. doi: 10.1186/s13008-023-00087-6. Cell Div. 2023. PMID: 37032375 Free PMC article. Review.
-
Isolation, purification and labeling of mouse bone marrow neutrophils for functional studies and adoptive transfer experiments.J Vis Exp. 2013 Jul 10;(77):e50586. doi: 10.3791/50586. J Vis Exp. 2013. PMID: 23892876 Free PMC article.
-
Gestation and breastfeeding in schistosomotic mice differentially alters the expression of histone deacetylases (HDACs) in adult offspring.Mem Inst Oswaldo Cruz. 2020 Feb 3;114:e190366. doi: 10.1590/0074-02760190366. eCollection 2020. Mem Inst Oswaldo Cruz. 2020. PMID: 32022099 Free PMC article.
-
Inhibitors of neutrophil recruitment identified using transgenic zebrafish to screen a natural product library.Dis Model Mech. 2014 Jan;7(1):163-9. doi: 10.1242/dmm.012047. Epub 2013 Nov 28. Dis Model Mech. 2014. PMID: 24291762 Free PMC article.
-
Phagocytosis of Necrotic Debris at Sites of Injury and Inflammation.Front Immunol. 2020 Jan 9;10:3030. doi: 10.3389/fimmu.2019.03030. eCollection 2019. Front Immunol. 2020. PMID: 31998312 Free PMC article. Review.
References
-
- Iijima M, Devreotes P. Tumor suppressor PTEN mediates sensing of chemoattractant gradients. Cell. 2002;109:599–610. - PubMed
-
- Funamoto S, Meili R, Lee S, Parry L, Firtel RA. Spatial and temporal regulation of 3-phosphoinositides by PI 3-kinase and PTEN mediates chemotaxis. Cell. 2002;109:611–623. - PubMed
-
- Li Z, et al. Regulation of PTEN by Rho small GTPases. Nat Cell Biol. 2005;7:399–404. - PubMed
-
- Li Z, et al. Directional sensing requires G beta gamma-mediated PAK1 and PIX alpha-dependent activation of Cdc42. Cell. 2003;114:215–227. - PubMed
-
- Chodniewicz D, Zhelev DV. Chemoattractant receptor-stimulated F-actin poly-merization in the human neutrophil is signaled by 2 distinct pathways. Blood. 2003;101:1181–1184. - PubMed
Publication types
MeSH terms
Substances
Grants and funding
LinkOut - more resources
Full Text Sources
Other Literature Sources
Molecular Biology Databases