The early autophagic pathway is activated by hepatitis B virus and required for viral DNA replication
- PMID: 20142477
- PMCID: PMC2840127
- DOI: 10.1073/pnas.0911373107
The early autophagic pathway is activated by hepatitis B virus and required for viral DNA replication
Abstract
Autophagy is a catabolic process by which cells remove long-lived proteins and damaged organelles for recycling. Viral infections may also induce autophagic response. Here we show that hepatitis B virus (HBV), a pathogen that chronically infects approximately 350 million people globally, can enhance autophagic response in cell cultures, mouse liver, and during natural infection. This enhancement of the autophagic response is not coupled by an increase of autophagic protein degradation and is dependent on the viral X protein, which binds to and enhances the enzymatic activity of phosphatidylinositol 3-kinase class III, an enzyme critical for the initiation of autophagy. Further analysis indicates that autophagy enhances HBV DNA replication, with minimal involvement of late autophagic vacuoles in this process. Our studies thus demonstrate that a DNA virus can use autophagy to enhance its own replication and indicate the possibility of targeting the autophagic pathway for the treatment of HBV patients.
Conflict of interest statement
The authors declare no conflict of interest.
Figures
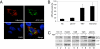
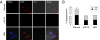
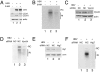
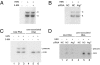
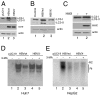
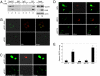
Similar articles
-
Glucosamine promotes hepatitis B virus replication through its dual effects in suppressing autophagic degradation and inhibiting MTORC1 signaling.Autophagy. 2020 Mar;16(3):548-561. doi: 10.1080/15548627.2019.1632104. Epub 2019 Jun 23. Autophagy. 2020. PMID: 31204557 Free PMC article.
-
PRKAA/AMPK restricts HBV replication through promotion of autophagic degradation.Autophagy. 2016 Sep;12(9):1507-20. doi: 10.1080/15548627.2016.1191857. Epub 2016 Jun 15. Autophagy. 2016. PMID: 27305174 Free PMC article.
-
Subversion of cellular autophagy machinery by hepatitis B virus for viral envelopment.J Virol. 2011 Jul;85(13):6319-33. doi: 10.1128/JVI.02627-10. Epub 2011 Apr 20. J Virol. 2011. PMID: 21507968 Free PMC article.
-
The role of HBV-induced autophagy in HBV replication and HBV related-HCC.Life Sci. 2018 Jul 15;205:107-112. doi: 10.1016/j.lfs.2018.04.051. Epub 2018 Apr 27. Life Sci. 2018. PMID: 29709654 Review.
-
Autophagy and microRNA in hepatitis B virus-related hepatocellular carcinoma.World J Gastroenterol. 2016 Jan 7;22(1):176-87. doi: 10.3748/wjg.v22.i1.176. World J Gastroenterol. 2016. PMID: 26755869 Free PMC article. Review.
Cited by
-
Replication of hepatitis C virus RNA on autophagosomal membranes.J Biol Chem. 2012 May 25;287(22):18036-43. doi: 10.1074/jbc.M111.320085. Epub 2012 Apr 10. J Biol Chem. 2012. PMID: 22496373 Free PMC article.
-
Autophagy Gene BECN1 Intronic Variant rs9890617 Predisposes Individuals to Hepatitis B Virus Infection.Biochem Genet. 2024 Oct;62(5):3336-3349. doi: 10.1007/s10528-023-10608-1. Epub 2023 Dec 16. Biochem Genet. 2024. PMID: 38103127
-
Autophagy is induced by swine acute diarrhea syndrome coronavirus through the cellular IRE1-JNK-Beclin 1 signaling pathway after an interaction of viral membrane-associated papain-like protease and GRP78.PLoS Pathog. 2023 Mar 8;19(3):e1011201. doi: 10.1371/journal.ppat.1011201. eCollection 2023 Mar. PLoS Pathog. 2023. PMID: 36888569 Free PMC article.
-
Human Papilloma Virus and Autophagy.Int J Mol Sci. 2018 Jun 15;19(6):1775. doi: 10.3390/ijms19061775. Int J Mol Sci. 2018. PMID: 29914057 Free PMC article. Review.
-
Autophagy Inhibits Grass Carp Reovirus (GCRV) Replication and Protects Ctenopharyngodon idella Kidney (CIK) Cells from Excessive Inflammatory Responses after GCRV Infection.Biomolecules. 2020 Sep 8;10(9):1296. doi: 10.3390/biom10091296. Biomolecules. 2020. PMID: 32911775 Free PMC article.
References
-
- Schmid D, Dengjel J, Schoor O, Stevanovic S, Münz C. Autophagy in innate and adaptive immunity against intracellular pathogens. J Mol Med. 2006;84:194–202. - PubMed
Publication types
MeSH terms
Substances
Grants and funding
LinkOut - more resources
Full Text Sources
Molecular Biology Databases