Synaptobrevin N-terminally bound to syntaxin-SNAP-25 defines the primed vesicle state in regulated exocytosis
- PMID: 20142423
- PMCID: PMC2819690
- DOI: 10.1083/jcb.200907018
Synaptobrevin N-terminally bound to syntaxin-SNAP-25 defines the primed vesicle state in regulated exocytosis
Abstract
Rapid neurotransmitter release depends on the ability to arrest the SNAP receptor (SNARE)-dependent exocytosis pathway at an intermediate "cocked" state, from which fusion can be triggered by Ca(2+). It is not clear whether this state includes assembly of synaptobrevin (the vesicle membrane SNARE) to the syntaxin-SNAP-25 (target membrane SNAREs) acceptor complex or whether the reaction is arrested upstream of that step. In this study, by a combination of in vitro biophysical measurements and time-resolved exocytosis measurements in adrenal chromaffin cells, we find that mutations of the N-terminal interaction layers of the SNARE bundle inhibit assembly in vitro and vesicle priming in vivo without detectable changes in triggering speed or fusion pore properties. In contrast, mutations in the last C-terminal layer decrease triggering speed and fusion pore duration. Between the two domains, we identify a region exquisitely sensitive to mutation, possibly constituting a switch. Our data are consistent with a model in which the N terminus of the SNARE complex assembles during vesicle priming, followed by Ca(2+)-triggered C-terminal assembly and membrane fusion.
Figures
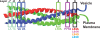
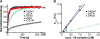
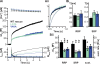
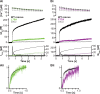
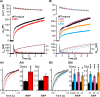
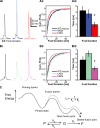
Similar articles
-
Sequential N- to C-terminal SNARE complex assembly drives priming and fusion of secretory vesicles.EMBO J. 2006 Mar 8;25(5):955-66. doi: 10.1038/sj.emboj.7601003. Epub 2006 Feb 23. EMBO J. 2006. PMID: 16498411 Free PMC article.
-
Sphingosine facilitates SNARE complex assembly and activates synaptic vesicle exocytosis.Neuron. 2009 Jun 11;62(5):683-94. doi: 10.1016/j.neuron.2009.04.024. Neuron. 2009. PMID: 19524527 Free PMC article.
-
Extension of Helix 12 in Munc18-1 Induces Vesicle Priming.J Neurosci. 2016 Jun 29;36(26):6881-91. doi: 10.1523/JNEUROSCI.0007-16.2016. J Neurosci. 2016. PMID: 27358447 Free PMC article.
-
Functional regulation of syntaxin-1: An underlying mechanism mediating exocytosis in neuroendocrine cells.Front Endocrinol (Lausanne). 2023 Jan 19;14:1096365. doi: 10.3389/fendo.2023.1096365. eCollection 2023. Front Endocrinol (Lausanne). 2023. PMID: 36742381 Free PMC article. Review.
-
Rab3 and synaptotagmin proteins in the regulation of vesicle fusion and neurotransmitter release.Life Sci. 2022 Nov 15;309:120995. doi: 10.1016/j.lfs.2022.120995. Epub 2022 Sep 24. Life Sci. 2022. PMID: 36167148 Review.
Cited by
-
Distinct Functions of Syntaxin-1 in Neuronal Maintenance, Synaptic Vesicle Docking, and Fusion in Mouse Neurons.J Neurosci. 2016 Jul 27;36(30):7911-24. doi: 10.1523/JNEUROSCI.1314-16.2016. J Neurosci. 2016. PMID: 27466336 Free PMC article.
-
Solution single-vesicle assay reveals PIP2-mediated sequential actions of synaptotagmin-1 on SNAREs.EMBO J. 2012 May 2;31(9):2144-55. doi: 10.1038/emboj.2012.57. Epub 2012 Mar 9. EMBO J. 2012. PMID: 22407297 Free PMC article.
-
Active zone scaffolds differentially accumulate Unc13 isoforms to tune Ca(2+) channel-vesicle coupling.Nat Neurosci. 2016 Oct;19(10):1311-20. doi: 10.1038/nn.4364. Epub 2016 Aug 15. Nat Neurosci. 2016. PMID: 27526206
-
CAPS and Munc13: CATCHRs that SNARE Vesicles.Front Endocrinol (Lausanne). 2013 Dec 4;4:187. doi: 10.3389/fendo.2013.00187. Front Endocrinol (Lausanne). 2013. PMID: 24363652 Free PMC article. Review.
-
Vesicle pools: lessons from adrenal chromaffin cells.Front Synaptic Neurosci. 2011 Feb 1;3:2. doi: 10.3389/fnsyn.2011.00002. eCollection 2011. Front Synaptic Neurosci. 2011. PMID: 21423410 Free PMC article.
References
-
- Ashery U., Betz A., Xu T., Brose N., Rettig J. 1999. An efficient method for infection of adrenal chromaffin cells using the Semliki Forest virus gene expression system. Eur. J. Cell Biol. 78:525–532 - PubMed
Publication types
MeSH terms
Substances
LinkOut - more resources
Full Text Sources
Other Literature Sources
Miscellaneous