Long-term potentiation depends on release of D-serine from astrocytes
- PMID: 20075918
- PMCID: PMC2807667
- DOI: 10.1038/nature08673
Long-term potentiation depends on release of D-serine from astrocytes
Abstract
Long-term potentiation (LTP) of synaptic transmission provides an experimental model for studying mechanisms of memory. The classical form of LTP relies on N-methyl-D-aspartate receptors (NMDARs), and it has been shown that astroglia can regulate their activation through Ca(2+)-dependent release of the NMDAR co-agonist D-serine. Release of D-serine from glia enables LTP in cultures and explains a correlation between glial coverage of synapses and LTP in the supraoptic nucleus. However, increases in Ca(2+) concentration in astroglia can also release other signalling molecules, most prominently glutamate, ATP and tumour necrosis factor-alpha, whereas neurons themselves can synthesize and supply D-serine. Furthermore, loading an astrocyte with exogenous Ca(2+) buffers does not suppress LTP in hippocampal area CA1 (refs 14-16), and the physiological relevance of experiments in cultures or strong exogenous stimuli applied to astrocytes has been questioned. The involvement of glia in LTP induction therefore remains controversial. Here we show that clamping internal Ca(2+) in individual CA1 astrocytes blocks LTP induction at nearby excitatory synapses by decreasing the occupancy of the NMDAR co-agonist sites. This LTP blockade can be reversed by exogenous D-serine or glycine, whereas depletion of D-serine or disruption of exocytosis in an individual astrocyte blocks local LTP. We therefore demonstrate that Ca(2+)-dependent release of D-serine from an astrocyte controls NMDAR-dependent plasticity in many thousands of excitatory synapses nearby.
Figures
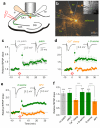
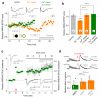
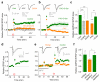
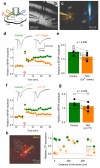
Comment in
-
Neuroscience: Astrocytes as aide-mémoires.Nature. 2010 Jan 14;463(7278):169-70. doi: 10.1038/463169a. Nature. 2010. PMID: 20075911 No abstract available.
Similar articles
-
Persistent activation of histamine H1 receptors in the hippocampal CA1 region enhances NMDA receptor-mediated synaptic excitation and long-term potentiation in astrocyte- and D-serine-dependent manner.Neuropharmacology. 2019 Jun;151:64-73. doi: 10.1016/j.neuropharm.2019.03.036. Epub 2019 Mar 31. Neuropharmacology. 2019. PMID: 30943384
-
A NMDA receptor glycine site partial agonist, GLYX-13, simultaneously enhances LTP and reduces LTD at Schaffer collateral-CA1 synapses in hippocampus.Neuropharmacology. 2008 Dec;55(7):1238-50. doi: 10.1016/j.neuropharm.2008.08.018. Epub 2008 Aug 29. Neuropharmacology. 2008. PMID: 18796308 Free PMC article.
-
Astrocytes mediate in vivo cholinergic-induced synaptic plasticity.PLoS Biol. 2012 Feb;10(2):e1001259. doi: 10.1371/journal.pbio.1001259. Epub 2012 Feb 14. PLoS Biol. 2012. PMID: 22347811 Free PMC article.
-
Hippocampal long-term synaptic plasticity and signal amplification of NMDA receptors.Crit Rev Neurobiol. 2006;18(1-2):71-84. doi: 10.1615/critrevneurobiol.v18.i1-2.80. Crit Rev Neurobiol. 2006. PMID: 17725510 Review.
-
Long-term potentiation and the role of N-methyl-D-aspartate receptors.Brain Res. 2015 Sep 24;1621:5-16. doi: 10.1016/j.brainres.2015.01.016. Epub 2015 Jan 22. Brain Res. 2015. PMID: 25619552 Free PMC article. Review.
Cited by
-
Drugs Based on NMDAR Hypofunction Hypothesis in Schizophrenia.Front Neurosci. 2021 Apr 12;15:641047. doi: 10.3389/fnins.2021.641047. eCollection 2021. Front Neurosci. 2021. PMID: 33912003 Free PMC article. Review.
-
Glial cells in Parkinson´s disease: protective or deleterious?Cell Mol Life Sci. 2020 Dec;77(24):5171-5188. doi: 10.1007/s00018-020-03584-x. Epub 2020 Jul 2. Cell Mol Life Sci. 2020. PMID: 32617639 Free PMC article. Review.
-
Differential sensitivity of brainstem versus cortical astrocytes to changes in pH reveals functional regional specialization of astroglia.J Neurosci. 2013 Jan 9;33(2):435-41. doi: 10.1523/JNEUROSCI.2813-12.2013. J Neurosci. 2013. PMID: 23303924 Free PMC article.
-
Storage and uptake of D-serine into astrocytic synaptic-like vesicles specify gliotransmission.J Neurosci. 2013 Feb 20;33(8):3413-23. doi: 10.1523/JNEUROSCI.3497-12.2013. J Neurosci. 2013. PMID: 23426669 Free PMC article.
-
Physical exercise enhances cognitive flexibility as well as astrocytic and synaptic markers in the medial prefrontal cortex.PLoS One. 2015 May 4;10(5):e0124859. doi: 10.1371/journal.pone.0124859. eCollection 2015. PLoS One. 2015. PMID: 25938418 Free PMC article.
References
REFERENCES (METHODS)
-
- Volterra A, Meldolesi J. Astrocytes, from brain glue to communication elements: the revolution continues. Nat Rev Neurosci. 2005;6:626–40. - PubMed
-
- Xu T, Binz T, Niemann H, Neher E. Multiple kinetic components of exocytosis distinguished by neurotoxin sensitivity. Nature Neurosci. 1998;1:192–200. - PubMed
-
- Szerb JC, Issekutz B. Increase in the stimulation-induced overflow of glutamate by fluoroacetate, a selective inhibitor of the glial tricarboxylic cycle. Brain Res. 1987;410:116–20. - PubMed
Publication types
MeSH terms
Substances
Grants and funding
LinkOut - more resources
Full Text Sources
Other Literature Sources
Miscellaneous