The clathrin heavy chain isoform CHC22 functions in a novel endosomal sorting step
- PMID: 20065094
- PMCID: PMC2812854
- DOI: 10.1083/jcb.200908057
The clathrin heavy chain isoform CHC22 functions in a novel endosomal sorting step
Abstract
Clathrin heavy chain 22 (CHC22) is an isoform of the well-characterized CHC17 clathrin heavy chain, a coat component of vesicles that mediate endocytosis and organelle biogenesis. CHC22 has a distinct role from CHC17 in trafficking glucose transporter 4 (GLUT4) in skeletal muscle and fat, though its transfection into HEK293 cells suggests functional redundancy. Here, we show that CHC22 is eightfold less abundant than CHC17 in muscle, other cell types have variably lower amounts of CHC22, and endogenous CHC22 and CHC17 function independently in nonmuscle and muscle cells. CHC22 was required for retrograde trafficking of certain cargo molecules from endosomes to the trans-Golgi network (TGN), defining a novel endosomal-sorting step distinguishable from that mediated by CHC17 and retromer. In muscle cells, depletion of syntaxin 10 as well as CHC22 affected GLUT4 targeting, establishing retrograde endosome-TGN transport as critical for GLUT4 trafficking. Like CHC22, syntaxin 10 is not expressed in mice but is present in humans and other vertebrates, implicating two species-restricted endosomal traffic proteins in GLUT4 transport.
Figures
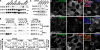
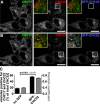
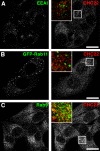
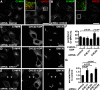
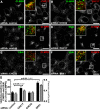
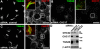
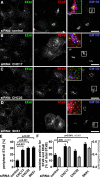
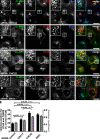
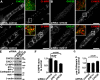
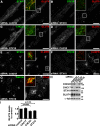
Similar articles
-
CHC22 clathrin recruitment to the early secretory pathway requires two-site interaction with SNX5 and p115.EMBO J. 2024 Oct;43(19):4298-4323. doi: 10.1038/s44318-024-00198-y. Epub 2024 Aug 19. EMBO J. 2024. PMID: 39160272 Free PMC article.
-
The CHC22 clathrin-GLUT4 transport pathway contributes to skeletal muscle regeneration.PLoS One. 2013 Oct 30;8(10):e77787. doi: 10.1371/journal.pone.0077787. eCollection 2013. PLoS One. 2013. PMID: 24204966 Free PMC article.
-
CHC22 and CHC17 clathrins have distinct biochemical properties and display differential regulation and function.J Biol Chem. 2017 Dec 22;292(51):20834-20844. doi: 10.1074/jbc.M117.816256. Epub 2017 Nov 2. J Biol Chem. 2017. PMID: 29097553 Free PMC article.
-
Building GLUT4 Vesicles: CHC22 Clathrin's Human Touch.Trends Cell Biol. 2020 Sep;30(9):705-719. doi: 10.1016/j.tcb.2020.05.007. Epub 2020 Jun 30. Trends Cell Biol. 2020. PMID: 32620516 Review.
-
Diversity of clathrin function: new tricks for an old protein.Annu Rev Cell Dev Biol. 2012;28:309-36. doi: 10.1146/annurev-cellbio-101011-155716. Epub 2012 Jul 23. Annu Rev Cell Dev Biol. 2012. PMID: 22831640 Review.
Cited by
-
Biogenesis and regulation of insulin-responsive vesicles containing GLUT4.Curr Opin Cell Biol. 2010 Aug;22(4):506-12. doi: 10.1016/j.ceb.2010.03.012. Epub 2010 Apr 21. Curr Opin Cell Biol. 2010. PMID: 20417083 Free PMC article. Review.
-
Single point mutations result in the miss-sorting of Glut4 to a novel membrane compartment associated with stress granule proteins.PLoS One. 2013 Jul 16;8(7):e68516. doi: 10.1371/journal.pone.0068516. Print 2013. PLoS One. 2013. PMID: 23874650 Free PMC article.
-
CHC22 clathrin recruitment to the early secretory pathway requires two-site interaction with SNX5 and p115.EMBO J. 2024 Oct;43(19):4298-4323. doi: 10.1038/s44318-024-00198-y. Epub 2024 Aug 19. EMBO J. 2024. PMID: 39160272 Free PMC article.
-
The CHC22 clathrin-GLUT4 transport pathway contributes to skeletal muscle regeneration.PLoS One. 2013 Oct 30;8(10):e77787. doi: 10.1371/journal.pone.0077787. eCollection 2013. PLoS One. 2013. PMID: 24204966 Free PMC article.
-
The retromer complex - endosomal protein recycling and beyond.J Cell Sci. 2012 Oct 15;125(Pt 20):4693-702. doi: 10.1242/jcs.103440. Epub 2012 Nov 12. J Cell Sci. 2012. PMID: 23148298 Free PMC article. Review.
References
-
- Banting G., Maile R., Roquemore E.P. 1998. The steady state distribution of humTGN46 is not significantly altered in cells defective in clathrin-mediated endocytosis. J. Cell Sci. 111:3451–3458 - PubMed
Publication types
MeSH terms
Substances
Grants and funding
LinkOut - more resources
Full Text Sources
Molecular Biology Databases
Miscellaneous