Prometaphase spindle maintenance by an antagonistic motor-dependent force balance made robust by a disassembling lamin-B envelope
- PMID: 20065089
- PMCID: PMC2812851
- DOI: 10.1083/jcb.200908150
Prometaphase spindle maintenance by an antagonistic motor-dependent force balance made robust by a disassembling lamin-B envelope
Abstract
We tested the classical hypothesis that astral, prometaphase bipolar mitotic spindles are maintained by balanced outward and inward forces exerted on spindle poles by kinesin-5 and -14 using modeling of in vitro and in vivo data from Drosophila melanogaster embryos. Throughout prometaphase, puncta of both motors aligned on interpolar microtubules (MTs [ipMTs]), and motor perturbation changed spindle length, as predicted. Competitive motility of purified kinesin-5 and -14 was well described by a stochastic, opposing power stroke model incorporating motor kinetics and load-dependent detachment. Motor parameters from this model were applied to a new stochastic force-balance model for prometaphase spindles, providing a good fit to data from embryos. Maintenance of virtual spindles required dynamic ipMTs and a narrow range of kinesin-5 to kinesin-14 ratios matching that found in embryos. Functional perturbation and modeling suggest that this range can be extended significantly by a disassembling lamin-B envelope that surrounds the prometaphase spindle and augments the finely tuned, antagonistic kinesin force balance to maintain robust prometaphase spindles as MTs assemble and chromosomes are pushed to the equator.
Figures
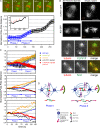
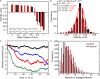
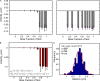
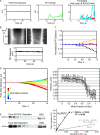
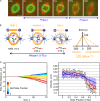
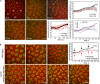
Similar articles
-
Kinesin-5-dependent poleward flux and spindle length control in Drosophila embryo mitosis.Mol Biol Cell. 2009 Mar;20(6):1749-62. doi: 10.1091/mbc.e08-10-1033. Epub 2009 Jan 21. Mol Biol Cell. 2009. PMID: 19158379 Free PMC article.
-
Microtubule flux and sliding in mitotic spindles of Drosophila embryos.Mol Biol Cell. 2002 Nov;13(11):3967-75. doi: 10.1091/mbc.02-05-0069. Mol Biol Cell. 2002. PMID: 12429839 Free PMC article.
-
Mechanism for Anaphase B: Evaluation of "Slide-and-Cluster" versus "Slide-and-Flux-or-Elongate" Models.Biophys J. 2015 Apr 21;108(8):2007-18. doi: 10.1016/j.bpj.2015.03.018. Biophys J. 2015. PMID: 25902440 Free PMC article.
-
Coupling between microtubule sliding, plus-end growth and spindle length revealed by kinesin-8 depletion.Cytoskeleton (Hoboken). 2010 Nov;67(11):715-28. doi: 10.1002/cm.20482. Cytoskeleton (Hoboken). 2010. PMID: 20814910 Free PMC article.
-
Mitotic functions of kinesin-5.Semin Cell Dev Biol. 2010 May;21(3):255-9. doi: 10.1016/j.semcdb.2010.01.019. Epub 2010 Jan 28. Semin Cell Dev Biol. 2010. PMID: 20109572 Free PMC article. Review.
Cited by
-
Mitotic membrane helps to focus and stabilize the mitotic spindle.Biophys J. 2010 Nov 17;99(10):3182-90. doi: 10.1016/j.bpj.2010.09.053. Biophys J. 2010. PMID: 21081065 Free PMC article.
-
Mechanisms by Which Kinesin-5 Motors Perform Their Multiple Intracellular Functions.Int J Mol Sci. 2021 Jun 15;22(12):6420. doi: 10.3390/ijms22126420. Int J Mol Sci. 2021. PMID: 34203964 Free PMC article. Review.
-
Physical determinants of bipolar mitotic spindle assembly and stability in fission yeast.Sci Adv. 2017 Jan 20;3(1):e1601603. doi: 10.1126/sciadv.1601603. eCollection 2017 Jan. Sci Adv. 2017. PMID: 28116355 Free PMC article.
-
The function of lamins in the context of tissue building and maintenance.Nucleus. 2012 May-Jun;3(3):256-62. doi: 10.4161/nucl.20392. Epub 2012 May 1. Nucleus. 2012. PMID: 22614537 Free PMC article.
-
Publishing and sharing multi-dimensional image data with OMERO.Mamm Genome. 2015 Oct;26(9-10):441-7. doi: 10.1007/s00335-015-9587-6. Epub 2015 Jul 30. Mamm Genome. 2015. PMID: 26223880 Free PMC article.
References
-
- Bray D. 2001. Cell Movements: From Molecules to Motility. Second edition Garland Pub., New York: 392
-
- Brust-Mascher I., Scholey J.M. 2009. Microinjection techniques for studying mitosis in the Drosophila melanogaster syncytial embryo. J. Vis. Exp. 31:http://www.jove.com/index/details.stp?id=1382 - PMC - PubMed
Publication types
MeSH terms
Substances
Grants and funding
LinkOut - more resources
Full Text Sources
Molecular Biology Databases
Miscellaneous