Jarid2/Jumonji coordinates control of PRC2 enzymatic activity and target gene occupancy in pluripotent cells
- PMID: 20064375
- PMCID: PMC2911953
- DOI: 10.1016/j.cell.2009.12.002
Jarid2/Jumonji coordinates control of PRC2 enzymatic activity and target gene occupancy in pluripotent cells
Abstract
Polycomb Repressive Complex 2 (PRC2) regulates key developmental genes in embryonic stem (ES) cells and during development. Here we show that Jarid2/Jumonji, a protein enriched in pluripotent cells and a founding member of the Jumonji C (JmjC) domain protein family, is a PRC2 subunit in ES cells. Genome-wide ChIP-seq analyses of Jarid2, Ezh2, and Suz12 binding reveal that Jarid2 and PRC2 occupy the same genomic regions. We further show that Jarid2 promotes PRC2 recruitment to the target genes while inhibiting PRC2 histone methyltransferase activity, suggesting that it acts as a "molecular rheostat" that finely calibrates PRC2 functions at developmental genes. Using Xenopus laevis as a model we demonstrate that Jarid2 knockdown impairs the induction of gastrulation genes in blastula embryos and results in failure of differentiation. Our findings illuminate a mechanism of histone methylation regulation in pluripotent cells and during early cell-fate transitions.
Copyright 2009 Elsevier Inc. All rights reserved.
Figures
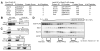
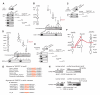
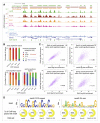
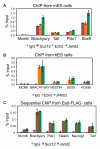
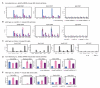
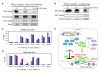
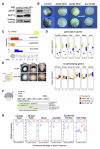
Similar articles
-
Jarid2 and PRC2, partners in regulating gene expression.Genes Dev. 2010 Feb 15;24(4):368-80. doi: 10.1101/gad.1886410. Epub 2010 Feb 1. Genes Dev. 2010. PMID: 20123894 Free PMC article.
-
PRC2 complexes with JARID2, MTF2, and esPRC2p48 in ES cells to modulate ES cell pluripotency and somatic cell reprogramming.Stem Cells. 2011 Feb;29(2):229-40. doi: 10.1002/stem.578. Stem Cells. 2011. PMID: 21732481 Free PMC article.
-
JARID2 regulates binding of the Polycomb repressive complex 2 to target genes in ES cells.Nature. 2010 Mar 11;464(7286):306-10. doi: 10.1038/nature08788. Nature. 2010. PMID: 20075857
-
Polycomb repressive complex 2 in embryonic stem cells: an overview.Protein Cell. 2010 Dec;1(12):1056-62. doi: 10.1007/s13238-010-0142-7. Epub 2011 Jan 8. Protein Cell. 2010. PMID: 21213100 Free PMC article. Review.
-
The Role of Polycomb Proteins in Cell Lineage Commitment and Embryonic Development.Epigenomes. 2022 Aug 12;6(3):23. doi: 10.3390/epigenomes6030023. Epigenomes. 2022. PMID: 35997369 Free PMC article. Review.
Cited by
-
Chromatin-bound RNA and the neurobiology of psychiatric disease.Neuroscience. 2014 Apr 4;264:131-41. doi: 10.1016/j.neuroscience.2013.06.051. Epub 2013 Jul 3. Neuroscience. 2014. PMID: 23831425 Free PMC article. Review.
-
Phf19 links methylated Lys36 of histone H3 to regulation of Polycomb activity.Nat Struct Mol Biol. 2012 Dec;19(12):1257-65. doi: 10.1038/nsmb.2434. Epub 2012 Oct 28. Nat Struct Mol Biol. 2012. PMID: 23104054 Free PMC article.
-
Mesp1 patterns mesoderm into cardiac, hematopoietic, or skeletal myogenic progenitors in a context-dependent manner.Cell Stem Cell. 2013 May 2;12(5):587-601. doi: 10.1016/j.stem.2013.03.004. Cell Stem Cell. 2013. PMID: 23642367 Free PMC article.
-
NuRD-mediated deacetylation of H3K27 facilitates recruitment of Polycomb Repressive Complex 2 to direct gene repression.EMBO J. 2012 Feb 1;31(3):593-605. doi: 10.1038/emboj.2011.431. Epub 2011 Dec 2. EMBO J. 2012. PMID: 22139358 Free PMC article.
-
Timing of transcriptional quiescence during gametogenesis is controlled by global histone H3K4 demethylation.Dev Cell. 2012 Nov 13;23(5):1059-71. doi: 10.1016/j.devcel.2012.10.005. Epub 2012 Nov 1. Dev Cell. 2012. PMID: 23123093 Free PMC article.
References
-
- Barski A, Cuddapah S, Cui K, Roh TY, Schones DE, Wang Z, Wei G, Chepelev I, Zhao K. High-resolution profiling of histone methylations in the human genome. Cell. 2007;129:823–837. - PubMed
-
- Beissbarth T, Speed TP. GOstat: find statistically overrepresented Gene Ontologies within a group of genes. Bioinformatics. 2004;20:1464–1465. - PubMed
Publication types
MeSH terms
Substances
Grants and funding
LinkOut - more resources
Full Text Sources
Other Literature Sources
Molecular Biology Databases