Molecular details of Bax activation, oligomerization, and membrane insertion
- PMID: 20008353
- PMCID: PMC2825459
- DOI: 10.1074/jbc.M109.081539
Molecular details of Bax activation, oligomerization, and membrane insertion
Abstract
Bax and Bid are pro-apoptotic members of the Bcl-2 protein family. Upon cleavage by caspase-8, Bid activates Bax. Activated Bax inserts into the mitochondrial outer membrane forming oligomers which lead to membrane poration, release of cytochrome c, and apoptosis. The detailed mechanism of Bax activation and the topology and composition of the oligomers are still under debate. Here molecular details of Bax activation and oligomerization were obtained by application of several biophysical techniques, including atomic force microscopy, cryoelectron microscopy, and particularly electron paramagnetic resonance (EPR) spectroscopy performed on spin-labeled Bax. Incubation with detergents, reconstitution, and Bid-triggered insertion into liposomes were found to be effective in inducing Bax oligomerization. Bid was shown to activate Bax independently of the stoichiometric ratio, suggesting that Bid has a catalytic function and that the interaction with Bax is transient. The formation of a stable dimerization interface involving two Bcl-2 homology 3 (BH3) domains was found to be the nucleation event for Bax homo-oligomerization. Based on intermolecular distance determined by EPR, a model of six adjacent Bax molecules in the oligomer is presented where the hydrophobic hairpins (helices alpha5 and alpha6) are equally spaced in the membrane and the two BH3 domains are in close vicinity in the dimer interface, separated by >5 nm from the next BH3 pairs.
Figures
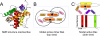
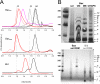
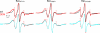
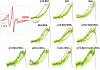
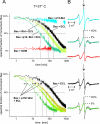
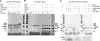
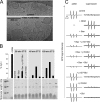
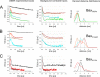
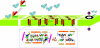
Similar articles
-
Humanin selectively prevents the activation of pro-apoptotic protein BID by sequestering it into fibers.J Biol Chem. 2020 Dec 25;295(52):18226-18238. doi: 10.1074/jbc.RA120.013023. Epub 2020 Oct 26. J Biol Chem. 2020. PMID: 33106313
-
Membrane-insertion fragments of Bcl-xL, Bax, and Bid.Biochemistry. 2004 Aug 31;43(34):10930-43. doi: 10.1021/bi036044c. Biochemistry. 2004. PMID: 15323553
-
Bcl-2 changes conformation to inhibit Bax oligomerization.EMBO J. 2006 Jun 7;25(11):2287-96. doi: 10.1038/sj.emboj.7601126. Epub 2006 Apr 27. EMBO J. 2006. PMID: 16642033 Free PMC article.
-
Structural biology of the Bcl-2 family of proteins.Biochim Biophys Acta. 2004 Mar 1;1644(2-3):83-94. doi: 10.1016/j.bbamcr.2003.08.012. Biochim Biophys Acta. 2004. PMID: 14996493 Review.
-
Bid: a Bax-like BH3 protein.Oncogene. 2008 Dec;27 Suppl 1:S93-104. doi: 10.1038/onc.2009.47. Oncogene. 2008. PMID: 19641510 Review.
Cited by
-
Pro-apoptotic Bax molecules densely populate the edges of membrane pores.Sci Rep. 2016 Jun 3;6:27299. doi: 10.1038/srep27299. Sci Rep. 2016. PMID: 27255832 Free PMC article.
-
In Situ Characterization of Bak Clusters Responsible for Cell Death Using Single Molecule Localization Microscopy.Sci Rep. 2016 Jun 13;6:27505. doi: 10.1038/srep27505. Sci Rep. 2016. PMID: 27293178 Free PMC article.
-
Bax activation initiates the assembly of a multimeric catalyst that facilitates Bax pore formation in mitochondrial outer membranes.PLoS Biol. 2012;10(9):e1001394. doi: 10.1371/journal.pbio.1001394. Epub 2012 Sep 25. PLoS Biol. 2012. PMID: 23049480 Free PMC article.
-
Parkin inhibits BAK and BAX apoptotic function by distinct mechanisms during mitophagy.EMBO J. 2019 Jan 15;38(2):e99916. doi: 10.15252/embj.201899916. Epub 2018 Dec 20. EMBO J. 2019. PMID: 30573668 Free PMC article.
-
RIPK3 mediates renal tubular epithelial cell apoptosis in endotoxin‑induced acute kidney injury.Mol Med Rep. 2019 Aug;20(2):1613-1620. doi: 10.3892/mmr.2019.10416. Epub 2019 Jun 24. Mol Med Rep. 2019. PMID: 31257491 Free PMC article.
References
-
- Cory S., Adams J. M. (2002) Nat. Rev. Cancer 2, 647–656 - PubMed
-
- Kuwana T., Newmeyer D. D. (2003) Curr. Opin. Cell Biol. 15, 691–699 - PubMed
-
- Li H., Zhu H., Xu C. J., Yuan J. (1998) Cell 94, 491–501 - PubMed
-
- Chipuk J. E., Bouchier-Hayes L., Green D. R. (2006) Cell Death Differ. 13, 1396–1402 - PubMed
-
- Kuwana T., Bouchier-Hayes L., Chipuk J. E., Bonzon C., Sullivan B. A., Green D. R., Newmeyer D. D. (2005) Mol. Cell 17, 525–535 - PubMed
Publication types
MeSH terms
Substances
LinkOut - more resources
Full Text Sources
Other Literature Sources
Research Materials