Langerhans cell (LC) proliferation mediates neonatal development, homeostasis, and inflammation-associated expansion of the epidermal LC network
- PMID: 19995948
- PMCID: PMC2806478
- DOI: 10.1084/jem.20091586
Langerhans cell (LC) proliferation mediates neonatal development, homeostasis, and inflammation-associated expansion of the epidermal LC network
Abstract
Most tissues develop from stem cells and precursors that undergo differentiation as their proliferative potential decreases. Mature differentiated cells rarely proliferate and are replaced at the end of their life by new cells derived from precursors. Langerhans cells (LCs) of the epidermis, although of myeloid origin, were shown to renew in tissues independently from the bone marrow, suggesting the existence of a dermal or epidermal progenitor. We investigated the mechanisms involved in LC development and homeostasis. We observed that a single wave of LC precursors was recruited in the epidermis of mice around embryonic day 18 and acquired a dendritic morphology, major histocompatibility complex II, CD11c, and langerin expression immediately after birth. Langerin(+) cells then undergo a massive burst of proliferation between postnatal day 2 (P2) and P7, expanding their numbers by 10-20-fold. After the first week of life, we observed low-level proliferation of langerin(+) cells within the epidermis. However, in a mouse model of atopic dermatitis (AD), a keratinocyte signal triggered increased epidermal LC proliferation. Similar findings were observed in epidermis from human patients with AD. Therefore, proliferation of differentiated resident cells represents an alternative pathway for development in the newborn, homeostasis, and expansion in adults of selected myeloid cell populations such as LCs. This mechanism may be relevant in locations where leukocyte trafficking is limited.
Figures
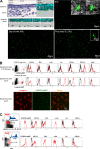
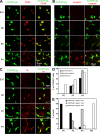
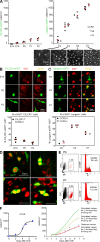
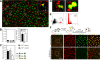
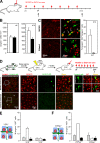
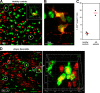
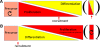
Similar articles
-
A model system using tape stripping for characterization of Langerhans cell-precursors in vivo.J Invest Dermatol. 2004 May;122(5):1165-74. doi: 10.1111/j.0022-202X.2004.22520.x. J Invest Dermatol. 2004. PMID: 15140219
-
The dermis contains langerin+ dendritic cells that develop and function independently of epidermal Langerhans cells.J Exp Med. 2007 Dec 24;204(13):3119-31. doi: 10.1084/jem.20071724. Epub 2007 Dec 17. J Exp Med. 2007. PMID: 18086861 Free PMC article.
-
Langerin-expressing dendritic cells in human tissues are related to CD1c+ dendritic cells and distinct from Langerhans cells and CD141high XCR1+ dendritic cells.J Leukoc Biol. 2015 Apr;97(4):627-34. doi: 10.1189/jlb.1HI0714-351R. Epub 2014 Dec 16. J Leukoc Biol. 2015. PMID: 25516751 Free PMC article.
-
Origin, homeostasis and function of Langerhans cells and other langerin-expressing dendritic cells.Nat Rev Immunol. 2008 Dec;8(12):935-47. doi: 10.1038/nri2455. Nat Rev Immunol. 2008. PMID: 19029989 Review.
-
[Langerhans cells in the physiopathology of atopic dermatitis].Ann Dermatol Venereol. 1990;117(3):185-93. Ann Dermatol Venereol. 1990. PMID: 2193589 Review. French.
Cited by
-
The role of Langerhans cells in epidermal homeostasis and pathogenesis of psoriasis.J Cell Mol Med. 2020 Oct;24(20):11646-11655. doi: 10.1111/jcmm.15834. Epub 2020 Sep 11. J Cell Mol Med. 2020. PMID: 32916775 Free PMC article. Review.
-
Regulation of macrophage and dendritic cell responses by their lineage precursors.J Innate Immun. 2012;4(5-6):411-23. doi: 10.1159/000335733. Epub 2012 Mar 16. J Innate Immun. 2012. PMID: 22433183 Free PMC article. Review.
-
Tissue-specific macrophages: how they develop and choreograph tissue biology.Nat Rev Immunol. 2023 Sep;23(9):563-579. doi: 10.1038/s41577-023-00848-y. Epub 2023 Mar 15. Nat Rev Immunol. 2023. PMID: 36922638 Free PMC article. Review.
-
Zinc in Keratinocytes and Langerhans Cells: Relevance to the Epidermal Homeostasis.J Immunol Res. 2018 Dec 9;2018:5404093. doi: 10.1155/2018/5404093. eCollection 2018. J Immunol Res. 2018. PMID: 30622978 Free PMC article. Review.
-
Langerhans cells are not required for graft-versus-host disease.Blood. 2011 Jan 13;117(2):697-707. doi: 10.1182/blood-2010-07-299073. Epub 2010 Oct 13. Blood. 2011. PMID: 20944073 Free PMC article.
References
-
- Alberts B., Johnson A., Lewis J., Raff M., Roberts K., Walter P. 2002. Molecular Biology of the Cell. 4th edition Garland Science, New York: 1616 pp
-
- Auffray C., Fogg D.K., Narni-Mancinelli E., Senechal B., Trouillet C., Saederup N., Leemput J., Bigot K., Campisi L., Abitbol M., et al. 2009. CX3CR1+ CD115+ CD135+ common macrophage/DC precursors and the role of CX3CR1 in their response to inflammation. J. Exp. Med. 206:595–606 10.1084/jem.20081385 - DOI - PMC - PubMed
Publication types
MeSH terms
Substances
Grants and funding
LinkOut - more resources
Full Text Sources
Other Literature Sources
Molecular Biology Databases
Research Materials