TACE antagonists blocking ACE2 shedding caused by the spike protein of SARS-CoV are candidate antiviral compounds
- PMID: 19995578
- PMCID: PMC7114272
- DOI: 10.1016/j.antiviral.2009.12.001
TACE antagonists blocking ACE2 shedding caused by the spike protein of SARS-CoV are candidate antiviral compounds
Abstract
Because outbreaks of severe acute respiratory syndrome coronavirus (SARS-CoV) might reemerge, identifying antiviral compounds is of key importance. Previously, we showed that the cellular factor TNF-alpha converting enzyme (TACE), activated by the spike protein of SARS-CoV (SARS-S protein), was positively involved in viral entry, implying that TACE is a possible target for developing antiviral compounds. To demonstrate this possibility, we here tested the effects of TACE inhibitors on viral entry. In vitro and in vivo data revealed that the TACE inhibitor TAPI-2 attenuated entry of both pseudotyped virus expressing the SARS-S protein in a lentiviral vector backbone and infectious SARS-CoV. TAPI-2 blocked both the SARS-S protein-induced shedding of angiotensin-converting enzyme 2 (ACE2), a receptor of SARS-CoV, and TNF-alpha production in lung tissues. Since the downregulation of ACE2 by SARS-S protein was proposed as an etiological event in the severe clinical manifestations, our data suggest that TACE antagonists block SARS-CoV infection and also attenuate its severe clinical outcome.
Figures
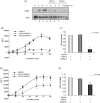
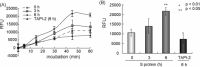
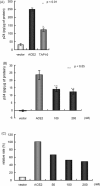
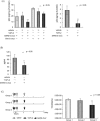
Similar articles
-
Modulation of TNF-alpha-converting enzyme by the spike protein of SARS-CoV and ACE2 induces TNF-alpha production and facilitates viral entry.Proc Natl Acad Sci U S A. 2008 Jun 3;105(22):7809-14. doi: 10.1073/pnas.0711241105. Epub 2008 May 19. Proc Natl Acad Sci U S A. 2008. PMID: 18490652 Free PMC article.
-
TMPRSS2 and ADAM17 cleave ACE2 differentially and only proteolysis by TMPRSS2 augments entry driven by the severe acute respiratory syndrome coronavirus spike protein.J Virol. 2014 Jan;88(2):1293-307. doi: 10.1128/JVI.02202-13. Epub 2013 Nov 13. J Virol. 2014. PMID: 24227843 Free PMC article.
-
Severe acute respiratory syndrome coronavirus entry as a target of antiviral therapies.Antivir Ther. 2007;12(4 Pt B):639-50. Antivir Ther. 2007. PMID: 17944271 Review.
-
Angiotensin-converting enzyme 2 (ACE2) from raccoon dog can serve as an efficient receptor for the spike protein of severe acute respiratory syndrome coronavirus.J Gen Virol. 2009 Nov;90(Pt 11):2695-2703. doi: 10.1099/vir.0.013490-0. Epub 2009 Jul 22. J Gen Virol. 2009. PMID: 19625462
-
The spike protein of SARS-CoV--a target for vaccine and therapeutic development.Nat Rev Microbiol. 2009 Mar;7(3):226-36. doi: 10.1038/nrmicro2090. Epub 2009 Feb 9. Nat Rev Microbiol. 2009. PMID: 19198616 Free PMC article. Review.
Cited by
-
Angiotensin-converting enzyme 2: the first decade.Int J Hypertens. 2012;2012:307315. doi: 10.1155/2012/307315. Epub 2011 Nov 10. Int J Hypertens. 2012. PMID: 22121476 Free PMC article.
-
Unraveling the Role of ACE2, the Binding Receptor for SARS-CoV-2, in Inflammatory Bowel Disease.Inflamm Bowel Dis. 2020 Nov 19;26(12):1787-1795. doi: 10.1093/ibd/izaa249. Inflamm Bowel Dis. 2020. PMID: 33064147 Free PMC article. Review.
-
Strategies for Targeting SARS CoV-2: Small Molecule Inhibitors-The Current Status.Front Immunol. 2020 Sep 18;11:552925. doi: 10.3389/fimmu.2020.552925. eCollection 2020. Front Immunol. 2020. PMID: 33072093 Free PMC article. Review.
-
Interactions between ibuprofen, ACE2, renin-angiotensin system, and spike protein in the lung. Implications for COVID-19.Clin Transl Med. 2021 Apr;11(4):e371. doi: 10.1002/ctm2.371. Clin Transl Med. 2021. PMID: 33931961 Free PMC article. No abstract available.
-
SARS-CoV-2 Evolutionary Adaptation toward Host Entry and Recognition of Receptor O-Acetyl Sialylation in Virus-Host Interaction.Int J Mol Sci. 2020 Jun 26;21(12):4549. doi: 10.3390/ijms21124549. Int J Mol Sci. 2020. PMID: 32604730 Free PMC article. Review.
References
-
- Black R.A., Rauch C.T., Kozlosky C.J., Peschon J.J., Slack J.L., Wolfson M.F., Castner B.J., Stocking K.L., Reddy P., Srinivasan S., Nelson N., Boiani N., Schooley K.A., Gerhart M., Davis R., Fitzner J.N., Johnson R.S., Paxton R.J., March C.J., Cerretti D.P. A metalloproteinase disintegrin that releases tumour-necrosis factor-α from cells. Nature. 1997;385:729–733. - PubMed
-
- Haga S., Yamamoto N., Nakai-Murakami C., Osawa Y., Tokunaga K., Sata T., Yamamoto N., Sasazuki T., Ishizaka Y. Modulation of TNF-α-converting enzyme by the spike protein of SARS-CoV and ACE2 induces TNF-α production and facilitates viral entry. Proc. Natl. Acad. Sci. U.S.A. 2008;105:7809–7814. - PMC - PubMed
-
- Jeffers S.A., Tusell S.M., Gillim-Ross L., Hemmila E.M., Achenbach J.E., Babcock G.J., Thomas W.D., Jr., Thackray L.B., Young M.D., Mason R.J., Ambrosino D.M., Wentworth D.E., Demartini J.C., Holmes K.V. CD209L (L-SIGN) is a receptor for severe acute respiratory syndrome coronavirus. Proc. Natl. Acad. Sci. U.S.A. 2004;101:15748–15753. - PMC - PubMed
-
- Ksiazek T.G., Erdman D., Goldsmith C.S., Zaki S.R., Peret T., Emery S., Tong S., Urbani C., Comer J.A., Lim W., Rollin P.E., Dowell S.F., Ling A.E., Humphrey C.D., Shieh W.J., Guarner J., Paddock C.D., Rota P., Fields B., DeRisi J., Yang J.Y., Cox N., Hughes J.M., LeDuc J.W., Bellini W.J., Anderson L.J., SARS Working Group A novel coronavirus associated with severe acute respiratory syndrome. N. Engl. J. Med. 2003;348:1953–1966. - PubMed
Publication types
MeSH terms
Substances
LinkOut - more resources
Full Text Sources
Other Literature Sources
Miscellaneous