Magnetic resonance neurography and diffusion tensor imaging: origins, history, and clinical impact of the first 50,000 cases with an assessment of efficacy and utility in a prospective 5000-patient study group
- PMID: 19927075
- PMCID: PMC2924821
- DOI: 10.1227/01.NEU.0000351279.78110.00
Magnetic resonance neurography and diffusion tensor imaging: origins, history, and clinical impact of the first 50,000 cases with an assessment of efficacy and utility in a prospective 5000-patient study group
Abstract
Objective: Methods were invented that made it possible to image peripheral nerves in the body and to image neural tracts in the brain. The history, physical basis, and dyadic tensor concept underlying the methods are reviewed. Over a 15-year period, these techniques-magnetic resonance neurography (MRN) and diffusion tensor imaging-were deployed in the clinical and research community in more than 2500 published research reports and applied to approximately 50,000 patients. Within this group, approximately 5000 patients having MRN were carefully tracked on a prospective basis.
Methods: A uniform Neurography imaging methodology was applied in the study group, and all images were reviewed and registered by referral source, clinical indication, efficacy of imaging, and quality. Various classes of image findings were identified and subjected to a variety of small targeted prospective outcome studies. Those findings demonstrated to be clinically significant were then tracked in the larger clinical volume data set.
Results: MRN demonstrates mechanical distortion of nerves, hyperintensity consistent with nerve irritation, nerve swelling, discontinuity, relations of nerves to masses, and image features revealing distortion of nerves at entrapment points. These findings are often clinically relevant and warrant full consideration in the diagnostic process. They result in specific pathological diagnoses that are comparable to electrodiagnostic testing in clinical efficacy. A review of clinical outcome studies with diffusion tensor imaging also shows convincing utility.
Conclusion: MRN and diffusion tensor imaging neural tract imaging have been validated as indispensable clinical diagnostic methods that provide reliable anatomic pathological information. There is no alternative diagnostic method in many situations. With the elapsing of 15 years, tens of thousands of imaging studies, and thousands of publications, these methods should no longer be considered experimental.
Figures
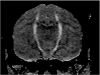
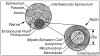
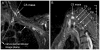
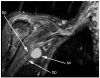
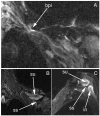
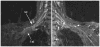
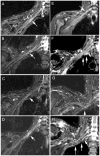
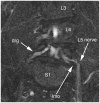
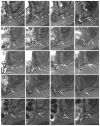
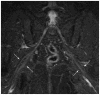
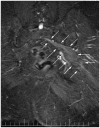
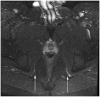
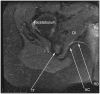
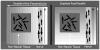
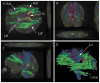
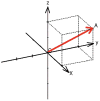
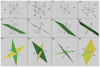
Similar articles
-
Magnetic resonance neurography: current perspectives and literature review.Eur Radiol. 2018 Feb;28(2):698-707. doi: 10.1007/s00330-017-4976-8. Epub 2017 Jul 14. Eur Radiol. 2018. PMID: 28710579 Review.
-
Magnetic resonance neurography and diffusion tensor imaging of the peripheral nerves in patients with Charcot-Marie-Tooth Type 1A.Muscle Nerve. 2017 Dec;56(6):E78-E84. doi: 10.1002/mus.25691. Epub 2017 May 29. Muscle Nerve. 2017. PMID: 28500667 Clinical Trial.
-
Diffusion-weighted MR neurography of median and ulnar nerves in the wrist and palm.Eur Radiol. 2017 Jun;27(6):2359-2366. doi: 10.1007/s00330-016-4591-0. Epub 2016 Sep 15. Eur Radiol. 2017. PMID: 27631109
-
Application of magnetic resonance neurography in the evaluation of patients with peripheral nerve pathology.J Neurosurg. 1996 Aug;85(2):299-309. doi: 10.3171/jns.1996.85.2.0299. J Neurosurg. 1996. PMID: 8755760
-
Current MR neurography techniques and whole-body MR neurography.Semin Musculoskelet Radiol. 2015 Apr;19(2):79-85. doi: 10.1055/s-0035-1545074. Epub 2015 Mar 12. Semin Musculoskelet Radiol. 2015. PMID: 25764231 Review.
Cited by
-
Opening up the window into "chemobrain": a neuroimaging review.Sensors (Basel). 2013 Mar 6;13(3):3169-203. doi: 10.3390/s130303169. Sensors (Basel). 2013. PMID: 23467031 Free PMC article. Review.
-
"Million dollar nerve" magnetic resonance neurography: first normal and pathological findings.Eur Radiol. 2022 Feb;32(2):1154-1162. doi: 10.1007/s00330-021-08213-0. Epub 2021 Aug 6. Eur Radiol. 2022. PMID: 34363135
-
Pectoralis Minor Syndrome: Subclavicular Brachial Plexus Compression.Diagnostics (Basel). 2017 Jul 28;7(3):46. doi: 10.3390/diagnostics7030046. Diagnostics (Basel). 2017. PMID: 28788065 Free PMC article. Review.
-
The Neurobiology of Eating Disorders.Child Adolesc Psychiatr Clin N Am. 2019 Oct;28(4):629-640. doi: 10.1016/j.chc.2019.05.007. Epub 2019 Jul 4. Child Adolesc Psychiatr Clin N Am. 2019. PMID: 31443880 Free PMC article. Review.
-
High-resolution 3D tractography of fibrous tissue based on polarization-sensitive optical coherence tomography.Exp Biol Med (Maywood). 2020 Feb;245(4):273-281. doi: 10.1177/1535370219894332. Epub 2019 Dec 8. Exp Biol Med (Maywood). 2020. PMID: 31813275 Free PMC article. Review.
References
-
- Aagaard BD, Maravilla KR, Kliot M. MR neurography. MR imaging of peripheral nerves. Magnetic resonance imaging clinics of North America. 1998;6:179–194. - PubMed
-
- Aagaard BD, Maravilla KR, Kliot M. Magnetic resonance neurography: magnetic resonance imaging of peripheral nerves. Neuroimaging clinics of North America. 2001;11:viii, 131–146. - PubMed
-
- Abrose J, Hounsfield G. Computerized transverse axial tomography. Br J Radiol. 1973;46:148–149. - PubMed
-
- Basser PJ, LeBihan D. Fiber orientation mapping in an anisotropic medium with NMR diffusion spectroscopy. Presented at Society for Magnetic Resonance in Medicine 11th Annual Meeting; Berlin, Germany. 1992.
Publication types
MeSH terms
Grants and funding
LinkOut - more resources
Full Text Sources
Other Literature Sources
Medical