10mM glucosamine prevents activation of proADAMTS5 (aggrecanase-2) in transfected cells by interference with post-translational modification of furin
- PMID: 19909832
- PMCID: PMC2826559
- DOI: 10.1016/j.joca.2009.10.014
10mM glucosamine prevents activation of proADAMTS5 (aggrecanase-2) in transfected cells by interference with post-translational modification of furin
Abstract
Objective: Glucosamine has been previously shown to suppress cartilage aggrecan catabolism in explant cultures. We determined the effect of glucosamine on ADAMTS5 (a disintegrin-like and metalloprotease domain (reprolysin type) with thrombospondin type-1 motifs 5), a major aggrecanase in osteoarthritis, and investigated a potential mechanism underlying the observed effects.
Design: HEK293F and CHO-K1 cells transiently transfected with ADAMTS5 cDNA were treated with glucosamine or the related hexosamine mannosamine. Glucosamine effects on FURIN transcription were determined by quantitative RT-PCR. Effects on furin-mediated processing of ADAMTS5 zymogen, and aggrecan processing by glucosamine-treated cells, were determined by western blotting. Post-translational modification of furin and N-glycan deficient furin mutants generated by site-directed mutagenesis was analyzed by western blotting, and the mutants were evaluated for their ADAMTS5 processing ability in furin-deficient CHO-RPE.40 cells.
Results: Ten mM glucosamine and 5-10mM mannosamine reduced excision of the ADAMTS5 propeptide, indicating interference with the propeptide excision mechanism, although mannosamine compromised cell viability at these doses. Although glucosamine had no effect on furin mRNA levels, western blot of furin from glucosamine-treated cells suggested altered post-translational modification. Glucosamine treatment led to decreased glycosylation of cellular furin, with reduced furin autoactivation as the consequence. Recombinant furin treated with peptide N-glycanase F had reduced activity against a synthetic peptide substrate. Indeed, site-directed mutagenesis of two furin N-glycosylation sites, Asn(387) and Asn(440), abrogated furin activation and this mutant was unable to rescue ADAMTS5 processing in furin-deficient cells.
Conclusions: Ten mM glucosamine reduces excision of the ADAMTS5 propeptide via interference with post-translational modification of furin and leads to reduced aggrecanase activity of ADAMTS5.
Copyright 2009 Osteoarthritis Research Society International. Published by Elsevier Ltd. All rights reserved.
Conflict of interest statement
None of the authors have any conflict of interest relating to the submitted manuscript.
Figures
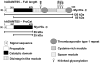
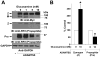
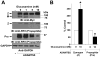
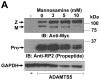
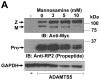
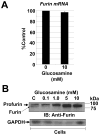
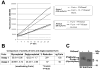
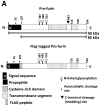
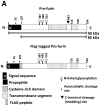
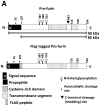
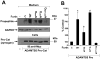
Similar articles
-
Characterization of proADAMTS5 processing by proprotein convertases.Int J Biochem Cell Biol. 2009 May;41(5):1116-26. doi: 10.1016/j.biocel.2008.10.008. Epub 2008 Nov 1. Int J Biochem Cell Biol. 2009. PMID: 18992360
-
Hsa-miR-15a exerts protective effects against osteoarthritis by targeting aggrecanase-2 (ADAMTS5) in human chondrocytes.Int J Mol Med. 2016 Feb;37(2):509-16. doi: 10.3892/ijmm.2015.2446. Epub 2015 Dec 23. Int J Mol Med. 2016. PMID: 26707794
-
Adamts5 (aggrecanase-2) is widely expressed in the mouse musculoskeletal system and is induced in specific regions of knee joint explants by inflammatory cytokines.J Orthop Res. 2012 Feb;30(2):226-33. doi: 10.1002/jor.21508. Epub 2011 Jul 28. J Orthop Res. 2012. PMID: 21800360
-
Anti-ADAMTS5 monoclonal antibodies: implications for aggrecanase inhibition in osteoarthritis.Biochem J. 2016 Jan 1;473(1):e1-4. doi: 10.1042/BJ20151072. Biochem J. 2016. PMID: 26657033 Free PMC article. Review.
-
Aggrecanase and aggrecan degradation in osteoarthritis: a review.J Int Med Res. 2008 Nov-Dec;36(6):1149-60. doi: 10.1177/147323000803600601. J Int Med Res. 2008. PMID: 19094423 Review.
Cited by
-
Glucosamine hydrochloride exerts a protective effect against unilateral ureteral obstruction-induced renal fibrosis by attenuating TGF-β signaling.J Mol Med (Berl). 2013 Nov;91(11):1273-84. doi: 10.1007/s00109-013-1086-1. Epub 2013 Sep 27. J Mol Med (Berl). 2013. PMID: 24072041 Free PMC article.
-
Increased Proteoglycanases in Pulmonary Valves after Birth Correlate with Extracellular Matrix Maturation and Valve Sculpting.J Cardiovasc Dev Dis. 2023 Jan 11;10(1):27. doi: 10.3390/jcdd10010027. J Cardiovasc Dev Dis. 2023. PMID: 36661922 Free PMC article.
-
Is there any scientific evidence for the use of glucosamine in the management of human osteoarthritis?Arthritis Res Ther. 2012 Jan 30;14(1):201. doi: 10.1186/ar3657. Arthritis Res Ther. 2012. PMID: 22293240 Free PMC article. Review.
-
Versican processing by a disintegrin-like and metalloproteinase domain with thrombospondin-1 repeats proteinases-5 and -15 facilitates myoblast fusion.J Biol Chem. 2013 Jan 18;288(3):1907-17. doi: 10.1074/jbc.M112.429647. Epub 2012 Dec 11. J Biol Chem. 2013. PMID: 23233679 Free PMC article.
-
Glucosamine and Silibinin Alter Cartilage Homeostasis through Glycosylation and Cellular Stresses in Human Chondrocyte Cells.Int J Mol Sci. 2024 Apr 30;25(9):4905. doi: 10.3390/ijms25094905. Int J Mol Sci. 2024. PMID: 38732122 Free PMC article.
References
-
- Pratta MA, Yao W, Decicco C, Tortorella MD, Liu RQ, Copeland RA, et al. Aggrecan protects cartilage collagen from proteolytic cleavage. J Biol Chem. 2003;278:45539–45545. - PubMed
-
- Abbaszade I, Liu RQ, Yang F, Rosenfeld SA, Ross OH, Link JR, et al. Cloning and characterization of ADAMTS11, an aggrecanase from the ADAMTS family. J Biol Chem. 1999;274:23443–23450. - PubMed
-
- Tortorella MD, Burn TC, Pratta MA, Abbaszade I, Hollis JM, Liu R, et al. Purification and cloning of aggrecanase-1: a member of the ADAMTS family of proteins [see comments] Science. 1999;284:1664–1666. - PubMed
-
- Fosang AJ, Little CB. Drug insight: aggrecanases as therapeutic targets for osteoarthritis. Nat Clin Pract Rheumatol. 2008;4:420–427. - PubMed
Publication types
MeSH terms
Substances
Grants and funding
LinkOut - more resources
Full Text Sources
Molecular Biology Databases