Blockade of Hsp20 phosphorylation exacerbates cardiac ischemia/reperfusion injury by suppressed autophagy and increased cell death
- PMID: 19850943
- PMCID: PMC2799045
- DOI: 10.1161/CIRCRESAHA.109.200378
Blockade of Hsp20 phosphorylation exacerbates cardiac ischemia/reperfusion injury by suppressed autophagy and increased cell death
Abstract
Rationale: The levels of a small heat shock protein (Hsp)20 and its phosphorylation are increased on ischemic insults, and overexpression of Hsp20 protects the heart against ischemia/reperfusion injury. However, the mechanism underlying cardioprotection of Hsp20 and especially the role of its phosphorylation in regulating ischemia/reperfusion-induced autophagy, apoptosis, and necrosis remain to be clarified.
Objective: Herein, we generated a cardiac-specific overexpression model, carrying nonphosphorylatable Hsp20, where serine 16 was substituted with alanine (Hsp20(S16A)). By subjecting this model to ischemia/reperfusion, we addressed whether: (1) the cardioprotective effects of Hsp20 are associated with serine 16 phosphorylation; (2) blockade of Hsp20 phosphorylation influences the balance between autophagy and cell death; and (3) the aggregation pattern of Hsp20 is altered by its phosphorylation.
Methods and results: Our results demonstrated that Hsp20(S16A) hearts were more sensitive to ischemia/reperfusion injury, evidenced by lower recovery of contractile function and increased necrosis and apoptosis, compared with non-TG hearts. Interestingly, autophagy was activated in non-TG hearts but significantly inhibited in Hsp20(S16A) hearts following ischemia/reperfusion. Accordingly, pretreatment of Hsp20(S16A) hearts with rapamycin, an activator of autophagy, resulted in improvement of functional recovery, compared with saline-treated Hsp20(S16A) hearts. Furthermore, on ischemia/reperfusion, the oligomerization pattern of Hsp20 appeared to shift to higher aggregates in Hsp20(S16A) hearts.
Conclusions: Collectively, these data indicate that blockade of Ser16-Hsp20 phosphorylation attenuates the cardioprotective effects of Hsp20 against ischemia/reperfusion injury, which may be attributable to suppressed autophagy and increased cell death. Therefore, phosphorylation of Hsp20 at serine 16 may represent a potential therapeutic target in ischemic heart disease.
Figures
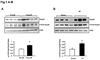
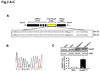
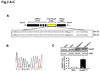
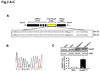
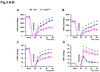
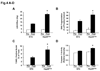
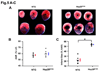
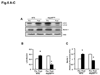
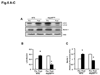
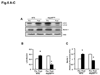
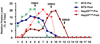
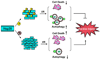
Similar articles
-
Novel cardioprotective role of a small heat-shock protein, Hsp20, against ischemia/reperfusion injury.Circulation. 2005 Apr 12;111(14):1792-9. doi: 10.1161/01.CIR.0000160851.41872.C6. Epub 2005 Apr 4. Circulation. 2005. PMID: 15809372
-
PKA phosphorylation of the small heat-shock protein Hsp20 enhances its cardioprotective effects.Biochem Soc Trans. 2012 Feb;40(1):210-4. doi: 10.1042/BST20110673. Biochem Soc Trans. 2012. PMID: 22260692 Free PMC article. Review.
-
Gene transfer of heat-shock protein 20 protects against ischemia/reperfusion injury in rat hearts.Acta Pharmacol Sin. 2005 Oct;26(10):1193-200. doi: 10.1111/j.1745-7254.2005.00139.x. Acta Pharmacol Sin. 2005. PMID: 16174435
-
Small heat shock protein 20 interacts with protein phosphatase-1 and enhances sarcoplasmic reticulum calcium cycling.Circ Res. 2011 Jun 10;108(12):1429-38. doi: 10.1161/CIRCRESAHA.110.237644. Epub 2011 Apr 14. Circ Res. 2011. PMID: 21493896 Free PMC article.
-
Hsp20 and its cardioprotection.Trends Cardiovasc Med. 2005 May;15(4):138-41. doi: 10.1016/j.tcm.2005.05.004. Trends Cardiovasc Med. 2005. PMID: 16099377 Review.
Cited by
-
Novel role of HAX-1 in ischemic injury protection involvement of heat shock protein 90.Circ Res. 2013 Jan 4;112(1):79-89. doi: 10.1161/CIRCRESAHA.112.279935. Epub 2012 Sep 14. Circ Res. 2013. PMID: 22982986 Free PMC article.
-
Autophagy in myocardium of murine hearts subjected to ischemia followed by reperfusion.Histochem Cell Biol. 2010 Nov;134(5):519-26. doi: 10.1007/s00418-010-0748-0. Epub 2010 Oct 8. Histochem Cell Biol. 2010. PMID: 20931339
-
Targeting sarcoplasmic reticulum calcium ATPase by gene therapy.Hum Gene Ther. 2013 Nov;24(11):937-47. doi: 10.1089/hum.2013.2512. Hum Gene Ther. 2013. PMID: 24164241 Free PMC article. Review.
-
Autophagy in Heart Failure: Insights into Mechanisms and Therapeutic Implications.J Cardiovasc Dev Dis. 2023 Aug 18;10(8):352. doi: 10.3390/jcdd10080352. J Cardiovasc Dev Dis. 2023. PMID: 37623365 Free PMC article. Review.
-
Nutrient-sensing mTORC1: Integration of metabolic and autophagic signals.J Mol Cell Cardiol. 2016 Jun;95:31-41. doi: 10.1016/j.yjmcc.2016.01.005. Epub 2016 Jan 7. J Mol Cell Cardiol. 2016. PMID: 26773603 Free PMC article. Review.
References
-
- Latchman DS. Heat shock proteins and cardiac protection. Cardiovasc Res. 2001;51:637–646. - PubMed
-
- Benjamin IJ, McMillan D. Stress (heat shock) proteins: molecular chaperones in cardiovascular biology and disease. Circ Res. 1998;83:117–132. - PubMed
-
- Vander Heide RS. Increased expression of HSP27 protects canine myocytes from simulated ischemia-reperfusion injury. Am J Physiol Heart Circ Physiol. 2002;282:H935–H941. - PubMed
-
- Morrison LE, Whittaker RJ, Klepper RE, Wawrousek EF, Glembotski CC. Roles for alphaB-crystallin and HSPB2 in protecting the myocardium from ischemia-reperfusion-induced damage in a KO mouse model. Am J Physiol Heart Circ Physiol. 2004;286:H847–H855. - PubMed
Publication types
MeSH terms
Substances
Grants and funding
- HL-26057/HL/NHLBI NIH HHS/United States
- HHMI/Howard Hughes Medical Institute/United States
- R01 HL087861-01/HL/NHLBI NIH HHS/United States
- R37 HL026057/HL/NHLBI NIH HHS/United States
- R01 HL087861/HL/NHLBI NIH HHS/United States
- R01 HL087861-03S1/HL/NHLBI NIH HHS/United States
- R01 HL087861-02/HL/NHLBI NIH HHS/United States
- HL-64018/HL/NHLBI NIH HHS/United States
- R01 HL026057/HL/NHLBI NIH HHS/United States
- HL-77101/HL/NHLBI NIH HHS/United States
- R01 HL064018/HL/NHLBI NIH HHS/United States
- R01 HL087861-03/HL/NHLBI NIH HHS/United States
- HL-087861/HL/NHLBI NIH HHS/United States
- P50 HL077101/HL/NHLBI NIH HHS/United States
LinkOut - more resources
Full Text Sources
Other Literature Sources
Medical
Molecular Biology Databases
Miscellaneous