CC chemokine receptor-1 activates intimal smooth muscle-like cells in graft arterial disease
- PMID: 19841301
- PMCID: PMC2996873
- DOI: 10.1161/CIRCULATIONAHA.109.859595
CC chemokine receptor-1 activates intimal smooth muscle-like cells in graft arterial disease
Abstract
Background: Graft arterial disease (GAD) limits long-term solid-organ allograft survival. The thickened intima in GAD contains smooth muscle-like cells (SMLCs), leukocytes, and extracellular matrix. The intimal SMLCs in mouse GAD lesions differ from medial smooth muscle cells in their function and phenotype. Although intimal SMLCs may originate by migration and modulation of donor medial cells or by recruitment of host-derived precursors, the mechanisms that underlie their localization within grafts and the factors that drive these processes remain unclear.
Methods and results: This study of aortic transplantation in mice demonstrated an important function for chemokines beyond their traditional role in leukocyte recruitment and activation. Intimal SMLCs, but not medial smooth muscle cells, express functional CC chemokine receptor-1 (CCR1) and respond to RANTES by increased migration and proliferation. Although RANTES infusion in vivo promoted inflammatory cell accumulation in the adventitia of aortic allografts of wild-type and CCR1-deficient recipients, it increased GAD intimal thickening with SMLC proliferation in only the wild-type hosts. Aortic allografts transplanted into CCR1-deficient mice after wild-type bone marrow transplantation did not develop intimal lesions, which indicates that CCR1-bearing inflammatory cells do not contribute to intimal lesion formation. Moreover, RANTES induced SMLC proliferation in vitro but did not promote medial smooth muscle cell growth. Blockade of CCR5 attenuated RANTES-induced T-cell and monocyte/macrophage proliferation but did not affect RANTES-induced SMLC proliferation, consistent with a larger role of CCR1-binding chemokines in SMLC migration and proliferation and GAD development.
Conclusions: These studies provide a novel mechanistic insight into the formation of vascular intimal hyperplasia and suggest a novel therapeutic strategy for preventing allograft arteriopathy.
Figures
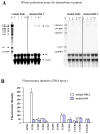
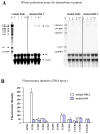
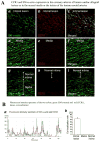
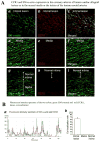
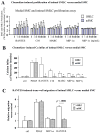
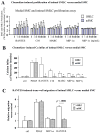
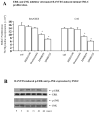
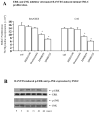
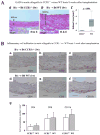
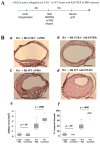
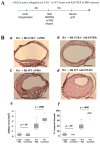
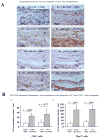
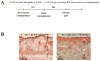
Comment in
-
Letter by Lee regarding article, "CC chemokine receptor-1 activates intimal smooth muscle-like cells in graft arterial disease".Circulation. 2010 Jun 15;121(23):e437; author reply e438. doi: 10.1161/CIRCULATIONAHA.109.921395. Circulation. 2010. PMID: 20547936 No abstract available.
Similar articles
-
Host bone-marrow cells are a source of donor intimal smooth- muscle-like cells in murine aortic transplant arteriopathy.Nat Med. 2001 Jun;7(6):738-41. doi: 10.1038/89121. Nat Med. 2001. PMID: 11385513
-
Inhibition of intimal hyperplasia in murine aortic allografts by the oral administration of the transforming growth factor-beta receptor I kinase inhibitor SD-208.J Heart Lung Transplant. 2014 Jun;33(6):654-61. doi: 10.1016/j.healun.2014.02.020. Epub 2014 Feb 21. J Heart Lung Transplant. 2014. PMID: 24685405
-
Inhibition of MCP-1/CCR2 signaling does not inhibit intimal proliferation in a mouse aortic transplant model.J Vasc Res. 2008;45(6):538-46. doi: 10.1159/000129688. Epub 2008 May 7. J Vasc Res. 2008. PMID: 18463419
-
Stem cell origins of intimal cells in graft arterial disease.Curr Atheroscler Rep. 2003 May;5(3):230-7. doi: 10.1007/s11883-003-0029-7. Curr Atheroscler Rep. 2003. PMID: 12667437 Review.
-
The role of progenitor cells in the development of intimal hyperplasia.J Vasc Surg. 2009 Feb;49(2):502-10. doi: 10.1016/j.jvs.2008.07.060. Epub 2008 Oct 22. J Vasc Surg. 2009. PMID: 18945574 Free PMC article. Review.
Cited by
-
Loss of myeloid related protein-8/14 exacerbates cardiac allograft rejection.Circulation. 2011 Dec 20;124(25):2920-32. doi: 10.1161/CIRCULATIONAHA.110.009910. Epub 2011 Dec 5. Circulation. 2011. PMID: 22144572 Free PMC article.
-
Genetic engineering of mesenchymal stem cells and its application in human disease therapy.Hum Gene Ther. 2010 Nov;21(11):1513-26. doi: 10.1089/hum.2010.165. Epub 2010 Oct 22. Hum Gene Ther. 2010. PMID: 20825283 Free PMC article. Review.
-
Protein targets of inflammatory serine proteases and cardiovascular disease.J Inflamm (Lond). 2010 Aug 30;7:45. doi: 10.1186/1476-9255-7-45. J Inflamm (Lond). 2010. PMID: 20804552 Free PMC article.
-
Genetic modification of mesenchymal stem cells overexpressing CCR1 increases cell viability, migration, engraftment, and capillary density in the injured myocardium.Circ Res. 2010 Jun 11;106(11):1753-62. doi: 10.1161/CIRCRESAHA.109.196030. Epub 2010 Apr 8. Circ Res. 2010. PMID: 20378860 Free PMC article.
References
-
- Libby P, Salomon RN, Payne DD, Schoen FJ, Pober JS. Functions of vascular wall cells related to development of transplantation-associated coronary arteriosclerosis. Transplant Proc. 1989;21:3677–3684. - PubMed
-
- Libby P, Pober JS. Chronic rejection. Immunity. 2001;14:387–397. - PubMed
-
- Kennedy LJ, Jr, Weissman IL. Dual origin of intimal cells in cardiac-allograft arteriosclerosis. N Engl J Med. 1971;285:884–887. - PubMed
-
- Shimizu K, Sugiyama S, Aikawa M, Fukumoto Y, Rabkin E, Libby P, Mitchell RN. Host bone-marrow cells are a source of donor intimal smooth- muscle-like cells in murine aortic transplant arteriopathy. Nat Med. 2001;7:738–741. - PubMed
-
- Shimizu K, Mitchell RN. Stem cell origins of intimal cells in graft arterial disease. Curr Atheroscler Rep. 2003;5:230–237. - PubMed
Publication types
MeSH terms
Substances
Grants and funding
- R01 HL067249-03/HL/NHLBI NIH HHS/United States
- R01 HL067283-04/HL/NHLBI NIH HHS/United States
- HL-67283/HL/NHLBI NIH HHS/United States
- HL-083154/HL/NHLBI NIH HHS/United States
- R37 HL034636/HL/NHLBI NIH HHS/United States
- R01 HL067283/HL/NHLBI NIH HHS/United States
- HL-43364/HL/NHLBI NIH HHS/United States
- R01 GM067049-04/GM/NIGMS NIH HHS/United States
- R01 HL043364-13/HL/NHLBI NIH HHS/United States
- HL-34636/HL/NHLBI NIH HHS/United States
- R01 HL067249/HL/NHLBI NIH HHS/United States
- R01 HL034636-20/HL/NHLBI NIH HHS/United States
- R37 HL034636-19/HL/NHLBI NIH HHS/United States
- R01 GM067049/GM/NIGMS NIH HHS/United States
- R01 HL034636/HL/NHLBI NIH HHS/United States
- R01 HL083154/HL/NHLBI NIH HHS/United States
- R01 HL043364-14/HL/NHLBI NIH HHS/United States
- R01 HL083154-03/HL/NHLBI NIH HHS/United States
- HL-67249/HL/NHLBI NIH HHS/United States
- R01 HL067283-03/HL/NHLBI NIH HHS/United States
- R01 GM067049-03/GM/NIGMS NIH HHS/United States
- R01 HL043364-15/HL/NHLBI NIH HHS/United States
- R01 HL067249-04/HL/NHLBI NIH HHS/United States
- GM-67049/GM/NIGMS NIH HHS/United States
LinkOut - more resources
Full Text Sources
Other Literature Sources
Molecular Biology Databases