AMP-activated protein kinase inhibits TREK channels
- PMID: 19840997
- PMCID: PMC2808542
- DOI: 10.1113/jphysiol.2009.180372
AMP-activated protein kinase inhibits TREK channels
Abstract
AMP-activated protein kinase (AMPK) is a serine/threonine kinase activated by conditions that increase the AMP : ATP ratio. In carotid body glomus cells, AMPK is thought to link changes in arterial O(2) with activation of glomus cells by inhibition of unidentified background K(+) channels. Modulation by AMPK of individual background K(+) channels has not been described. Here, we characterize effects of activated AMPK on recombinant TASK-1, TASK-3, TREK-1 and TREK-2 background K(+) channels expressed in HEK293 cells. We found that TREK-1 and TREK-2 channels but not TASK-1 or TASK-3 channels are inhibited by AMPK. AMPK-mediated inhibition of TREK involves key serine residues in the C-terminus that are also known to be important for PKA and PKC channel modulation; inhibition of TREK-1 requires Ser-300 and Ser-333 and inhibition of TREK-2 requires Ser-326 and Ser-359. Metabolic inhibition by sodium azide can also inhibit both TREK and TASK channels. The effects of azide on TREK occlude subsequent channel inhibition by AMPK and are attenuated by expression of a dominant negative catalytic subunit of AMPK (dnAMPK), suggesting that metabolic stress modulates TREK channels by an AMPK mechanism. By contrast, inhibition of TASK channels by azide was unaffected by expression of dnAMPK, suggesting an AMPK-independent mechanism. In addition, prolonged exposure (6-7 min) to hypoxia ( = 11 +/- 1 mmHg) inhibits TREK channels and this response was blocked by expression of dnAMPK. Our results identify a novel modulation of TREK channels by AMPK and indicate that select residues in the C-terminus of TREK are points of convergence for multiple signalling cascades including AMPK, PKA and PKC. To the extent that carotid body O(2) sensitivity is dependent on AMPK, our finding that TREK-1 and TREK-2 channels are inhibited by AMPK suggests that TREK channels may represent the AMPK-inhibited background K(+) channels that mediate activation of glomus cells by hypoxia.
Figures
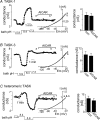
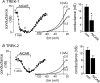
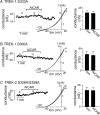
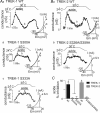
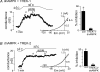
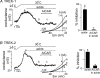
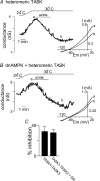
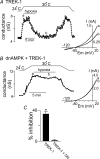


Similar articles
-
Sequential phosphorylation mediates receptor- and kinase-induced inhibition of TREK-1 background potassium channels.J Biol Chem. 2005 Aug 26;280(34):30175-84. doi: 10.1074/jbc.M503862200. Epub 2005 Jul 8. J Biol Chem. 2005. PMID: 16006563
-
Modulation of O(2) sensitive K (+) channels by AMP-activated protein kinase.Adv Exp Med Biol. 2009;648:57-63. doi: 10.1007/978-90-481-2259-2_6. Adv Exp Med Biol. 2009. PMID: 19536465
-
Effects of modulators of AMP-activated protein kinase on TASK-1/3 and intracellular Ca(2+) concentration in rat carotid body glomus cells.Respir Physiol Neurobiol. 2014 May 1;195:19-26. doi: 10.1016/j.resp.2014.01.020. Epub 2014 Feb 13. Respir Physiol Neurobiol. 2014. PMID: 24530802 Free PMC article.
-
Ion channel regulation by the LKB1-AMPK signalling pathway: the key to carotid body activation by hypoxia and metabolic homeostasis at the whole body level.Adv Exp Med Biol. 2012;758:81-90. doi: 10.1007/978-94-007-4584-1_11. Adv Exp Med Biol. 2012. PMID: 23080146 Review.
-
TREK-1 K(+) channels in the cardiovascular system: their significance and potential as a therapeutic target.Cardiovasc Ther. 2012 Feb;30(1):e23-9. doi: 10.1111/j.1755-5922.2010.00227.x. Epub 2010 Oct 14. Cardiovasc Ther. 2012. PMID: 20946320 Review.
Cited by
-
The human carotid body transcriptome with focus on oxygen sensing and inflammation--a comparative analysis.J Physiol. 2012 Aug 15;590(16):3807-19. doi: 10.1113/jphysiol.2012.231084. Epub 2012 May 21. J Physiol. 2012. PMID: 22615433 Free PMC article.
-
Are TREK Channels Temperature Sensors?Front Cell Neurosci. 2021 Oct 6;15:744702. doi: 10.3389/fncel.2021.744702. eCollection 2021. Front Cell Neurosci. 2021. PMID: 34690704 Free PMC article. Review.
-
Altered detrusor contractility and voiding patterns in mice lacking the mechanosensitive TREK-1 channel.BMC Urol. 2019 May 21;19(1):40. doi: 10.1186/s12894-019-0475-3. BMC Urol. 2019. PMID: 31113422 Free PMC article.
-
Tandem pore TWIK-related potassium channels and neuroprotection.Neural Regen Res. 2019 Aug;14(8):1293-1308. doi: 10.4103/1673-5374.253506. Neural Regen Res. 2019. PMID: 30964046 Free PMC article. Review.
-
Tubulin binds to the cytoplasmic loop of TRESK background K⁺ channel in vitro.PLoS One. 2014 May 15;9(5):e97854. doi: 10.1371/journal.pone.0097854. eCollection 2014. PLoS One. 2014. PMID: 24830385 Free PMC article.
References
-
- Buckler KJ. TASK-like potassium channels and oxygen sensing in the carotid body. Respir Physiol Neurobiol. 2007;157:55–64. - PubMed
Publication types
MeSH terms
Substances
Grants and funding
LinkOut - more resources
Full Text Sources