The annealing helicase SMARCAL1 maintains genome integrity at stalled replication forks
- PMID: 19793861
- PMCID: PMC2764496
- DOI: 10.1101/gad.1839909
The annealing helicase SMARCAL1 maintains genome integrity at stalled replication forks
Abstract
Mutations in SMARCAL1 (HARP) cause Schimke immunoosseous dysplasia (SIOD). The mechanistic basis for this disease is unknown. Using functional genomic screens, we identified SMARCAL1 as a genome maintenance protein. Silencing and overexpression of SMARCAL1 leads to activation of the DNA damage response during S phase in the absence of any genotoxic agent. SMARCAL1 contains a Replication protein A (RPA)-binding motif similar to that found in the replication stress response protein TIPIN (Timeless-Interacting Protein), which is both necessary and sufficient to target SMARCAL1 to stalled replication forks. RPA binding is critical for the cellular function of SMARCAL1; however, it is not necessary for the annealing helicase activity of SMARCAL1 in vitro. An SIOD-associated SMARCAL1 mutant fails to prevent replication-associated DNA damage from accumulating in cells in which endogenous SMARCAL1 is silenced. Ataxia-telangiectasia mutated (ATM), ATM and Rad3-related (ATR), and DNA-dependent protein kinase (DNA-PK) phosphorylate SMARCAL1 in response to replication stress. Loss of SMARCAL1 activity causes increased RPA loading onto chromatin and persistent RPA phosphorylation after a transient exposure to replication stress. Furthermore, SMARCAL1-deficient cells are hypersensitive to replication stress agents. Thus, SMARCAL1 is a replication stress response protein, and the pleiotropic phenotypes of SIOD are at least partly due to defects in genome maintenance during DNA replication.
Figures
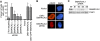
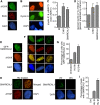
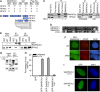
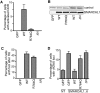
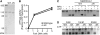
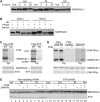
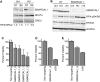
Comment in
-
HARPing on about the DNA damage response during replication.Genes Dev. 2009 Oct 15;23(20):2359-65. doi: 10.1101/gad.1860609. Genes Dev. 2009. PMID: 19833762 Free PMC article.
Similar articles
-
SMARCAL1 and replication stress: an explanation for SIOD?Nucleus. 2010 May-Jun;1(3):245-8. doi: 10.4161/nucl.1.3.11739. Epub 2010 Feb 16. Nucleus. 2010. PMID: 21327070 Free PMC article.
-
The SIOD disorder protein SMARCAL1 is an RPA-interacting protein involved in replication fork restart.Genes Dev. 2009 Oct 15;23(20):2415-25. doi: 10.1101/gad.1832309. Epub 2009 Sep 30. Genes Dev. 2009. PMID: 19793862 Free PMC article.
-
[SMARCAL1, roles and mechanisms in genome stability maintenance].Yi Chuan. 2019 Dec 20;41(12):1084-1098. doi: 10.16288/j.yczz.19-158. Yi Chuan. 2019. PMID: 31857280 Review. Chinese.
-
The annealing helicase HARP protects stalled replication forks.Genes Dev. 2009 Oct 15;23(20):2394-9. doi: 10.1101/gad.1836409. Epub 2009 Sep 30. Genes Dev. 2009. PMID: 19793864 Free PMC article.
-
The role of SMARCAL1 in replication fork stability and telomere maintenance.DNA Repair (Amst). 2017 Aug;56:129-134. doi: 10.1016/j.dnarep.2017.06.015. Epub 2017 Jun 10. DNA Repair (Amst). 2017. PMID: 28623093 Review.
Cited by
-
Replication stress in Mammalian cells and its consequences for mitosis.Genes (Basel). 2015 May 22;6(2):267-98. doi: 10.3390/genes6020267. Genes (Basel). 2015. PMID: 26010955 Free PMC article. Review.
-
Homologous recombination defects and how they affect replication fork maintenance.AIMS Genet. 2019 Apr 3;5(4):192-211. doi: 10.3934/genet.2018.4.192. eCollection 2018. AIMS Genet. 2019. PMID: 31435521 Free PMC article. Review.
-
E3 ligase RFWD3 participates in replication checkpoint control.J Biol Chem. 2011 Jun 24;286(25):22308-13. doi: 10.1074/jbc.M111.222869. Epub 2011 Apr 18. J Biol Chem. 2011. PMID: 21504906 Free PMC article.
-
hPrimpol1/CCDC111 is a human DNA primase-polymerase required for the maintenance of genome integrity.EMBO Rep. 2013 Dec;14(12):1104-12. doi: 10.1038/embor.2013.159. Epub 2013 Oct 15. EMBO Rep. 2013. PMID: 24126761 Free PMC article.
-
Simultaneous binding to the tracking strand, displaced strand and the duplex of a DNA fork enhances unwinding by Dda helicase.Nucleic Acids Res. 2014 Oct;42(18):11707-20. doi: 10.1093/nar/gku845. Epub 2014 Sep 23. Nucleic Acids Res. 2014. PMID: 25249618 Free PMC article.
References
-
- Abraham RT. PI 3-kinase related kinases: ‘Big’ players in stress-induced signaling pathways. DNA Repair (Amst) 2004;3:883–887. - PubMed
-
- Bartkova J, Horejsi Z, Koed K, Kramer A, Tort F, Zieger K, Guldberg P, Sehested M, Nesland JM, Lukas C, et al. DNA damage response as a candidate anti-cancer barrier in early human tumorigenesis. Nature. 2005;434:864–870. - PubMed
-
- Bartkova J, Rezaei N, Liontos M, Karakaidos P, Kletsas D, Issaeva N, Vassiliou LV, Kolettas E, Niforou K, Zoumpourlis VC, et al. Oncogene-induced senescence is part of the tumorigenesis barrier imposed by DNA damage checkpoints. Nature. 2006;444:633–637. - PubMed
-
- Barzilai A, Biton S, Shiloh Y. The role of the DNA damage response in neuronal development, organization and maintenance. DNA Repair (Amst) 2008;7:1010–1027. - PubMed
Publication types
MeSH terms
Substances
Grants and funding
LinkOut - more resources
Full Text Sources
Molecular Biology Databases
Research Materials
Miscellaneous