CTF determination and correction for low dose tomographic tilt series
- PMID: 19732834
- PMCID: PMC2784817
- DOI: 10.1016/j.jsb.2009.08.016
CTF determination and correction for low dose tomographic tilt series
Abstract
The resolution of cryo-electron tomography can be limited by the first zero of the microscope's contrast transfer function (CTF). To achieve higher resolution, it is critical to determine the CTF and correct its phase inversions. However, the extremely low signal-to-noise ratio (SNR) and the defocus gradient in the projections of tilted specimens make this process challenging. Two programs, CTFPLOTTER and CTFPHASEFLIP, have been developed to address these issues. CTFPLOTTER obtains a 1D power spectrum by periodogram averaging and rotational averaging and it estimates the noise background with a novel approach, which uses images taken with no specimen. The background-subtracted 1D power spectra from image regions at different defocus values are then shifted to align their first zeros and averaged together. This averaging improves the SNR sufficiently that it becomes possible to determine the defocus for subsets of the tilt series rather than just the entire series. CTFPHASEFLIP corrects images line-by-line by inverting phases appropriately in thin strips of the image at nearly constant defocus. CTF correction by these methods is shown to improve the resolution of aligned, averaged particles extracted from tomograms. However, some restoration of Fourier amplitudes at high frequencies is important for seeing the benefits from CTF correction.
Figures
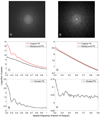
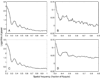
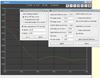
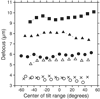
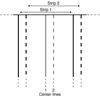
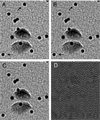
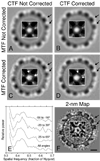
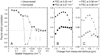
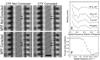
Similar articles
-
Contrast transfer function correction applied to cryo-electron tomography and sub-tomogram averaging.J Struct Biol. 2009 Nov;168(2):305-12. doi: 10.1016/j.jsb.2009.08.002. Epub 2009 Aug 8. J Struct Biol. 2009. PMID: 19666126 Free PMC article.
-
Tilt-series-based joint CTF estimation for cryo-electron tomography.Structure. 2024 Aug 8;32(8):1239-1247.e3. doi: 10.1016/j.str.2024.05.006. Epub 2024 May 31. Structure. 2024. PMID: 38823380
-
Alignment algorithms and per-particle CTF correction for single particle cryo-electron tomography.J Struct Biol. 2016 Jun;194(3):383-94. doi: 10.1016/j.jsb.2016.03.018. Epub 2016 Mar 22. J Struct Biol. 2016. PMID: 27016284 Free PMC article.
-
Cryo-Electron Tomography and Subtomogram Averaging.Methods Enzymol. 2016;579:329-67. doi: 10.1016/bs.mie.2016.04.014. Epub 2016 Jun 22. Methods Enzymol. 2016. PMID: 27572733 Review.
-
High-resolution cryo-electron microscopy on macromolecular complexes and cell organelles.Protoplasma. 2014 Mar;251(2):417-27. doi: 10.1007/s00709-013-0600-1. Epub 2014 Jan 5. Protoplasma. 2014. PMID: 24390311 Free PMC article. Review.
Cited by
-
Electron Tomography: A Three-Dimensional Analytic Tool for Hard and Soft Materials Research.Adv Mater. 2015 Oct 14;27(38):5638-63. doi: 10.1002/adma.201501015. Epub 2015 Jun 18. Adv Mater. 2015. PMID: 26087941 Free PMC article. Review.
-
Cryo electron tomography with volta phase plate reveals novel structural foundations of the 96-nm axonemal repeat in the pathogen Trypanosoma brucei.Elife. 2019 Nov 11;8:e52058. doi: 10.7554/eLife.52058. Elife. 2019. PMID: 31710293 Free PMC article.
-
Electron cryotomography analysis of Dam1C/DASH at the kinetochore-spindle interface in situ.J Cell Biol. 2019 Feb 4;218(2):455-473. doi: 10.1083/jcb.201809088. Epub 2018 Nov 30. J Cell Biol. 2019. PMID: 30504246 Free PMC article.
-
Organisation of the orthobunyavirus tripodal spike and the structural changes induced by low pH and K+ during entry.Nat Commun. 2023 Sep 21;14(1):5885. doi: 10.1038/s41467-023-41205-w. Nat Commun. 2023. PMID: 37735161 Free PMC article.
-
Structure of the immature HIV-1 capsid in intact virus particles at 8.8 Å resolution.Nature. 2015 Jan 22;517(7535):505-8. doi: 10.1038/nature13838. Epub 2014 Nov 2. Nature. 2015. PMID: 25363765
References
-
- Zhu J, Penczek PA, Schroder R, Frank J. Three-dimensional reconstruction with contrast transfer function correction from energy-filtered cryoelectron micrographs: procedure and application to the 70S Escherichia coli ribosome. J. Struct. Biol. 1997;118:197–219. - PubMed
-
- Toyoshima C, Unwin N. Contrast transfer for frozen-hydrated specimens: determination from pairs of defocused images. Ultramicroscopy. 1988;25:279–291. - PubMed
-
- Thon F. Zur Defokussierungsabhangigkeit des Phasenkontrastes bei der elektronenmikroskopischen Abbildung. Z. Naturforshung. 1966:476–478.
-
- Henderson R, Baldwin JM, Downing KH, Lepault J, Zemlin F. Structure of purple membrane from halobacterium-halobium, recording, measurement and evaluation of electron-micrographs at 3.5 Å resolution. Ultramicroscopy. 1986;19:147–178.
-
- Fernandez J-J, Sanjurjo JR, Carazo J-M. A spectral estimation approach to contrast transfer function detection in electron microscopy. Ultramicroscopy. 1997;68:267–295.
Publication types
MeSH terms
Grants and funding
LinkOut - more resources
Full Text Sources