Phosphorylation of caveolin-1 regulates oxidant-induced pulmonary vascular permeability via paracellular and transcellular pathways
- PMID: 19713536
- PMCID: PMC2776728
- DOI: 10.1161/CIRCRESAHA.109.201673
Phosphorylation of caveolin-1 regulates oxidant-induced pulmonary vascular permeability via paracellular and transcellular pathways
Abstract
Rationale: Oxidants are important signaling molecules known to increase endothelial permeability, although the mechanisms underlying permeability regulation are not clear.
Objective: To define the role of caveolin-1 in the mechanism of oxidant-induced pulmonary vascular hyperpermeability and edema formation.
Methods and results: Using genetic approaches, we show that phosphorylation of caveolin-1 Tyr14 is required for increased pulmonary microvessel permeability induced by hydrogen peroxide (H(2)O(2)). Caveolin-1-deficient mice (cav-1(-/-)) were resistant to H(2)O(2)-induced pulmonary vascular albumin hyperpermeability and edema formation. Furthermore, the vascular hyperpermeability response to H(2)O(2) was completely rescued by expression of caveolin-1 in cav-1(-/-) mouse lung microvessels but was not restored by the phosphorylation-defective caveolin-1 mutant. The increase in caveolin-1 phosphorylation induced by H(2)O(2) was dose-dependently coupled to both increased (125)I-albumin transcytosis and decreased transendothelial electric resistance in pulmonary endothelial cells. Phosphorylation of caveolin-1 following H(2)O(2) exposure resulted in the dissociation of vascular endothelial cadherin/beta-catenin complexes and resultant endothelial barrier disruption.
Conclusions: Caveolin-1 phosphorylation-dependent signaling plays a crucial role in oxidative stress-induced pulmonary vascular hyperpermeability via transcellular and paracellular pathways. Thus, caveolin-1 phosphorylation may be an important therapeutic target for limiting oxidant-mediated vascular hyperpermeability, protein-rich edema formation, and acute lung injury.
Conflict of interest statement
Figures
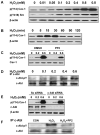
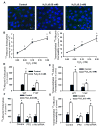
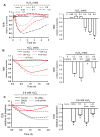
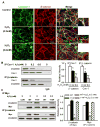
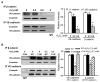
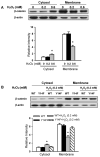
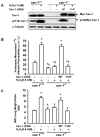
Similar articles
-
Lipopolysaccharide-induced caveolin-1 phosphorylation-dependent increase in transcellular permeability precedes the increase in paracellular permeability.Drug Des Devel Ther. 2015 Aug 28;9:4965-77. doi: 10.2147/DDDT.S77646. eCollection 2015. Drug Des Devel Ther. 2015. PMID: 26357463 Free PMC article.
-
Intercellular adhesion molecule-1-dependent neutrophil adhesion to endothelial cells induces caveolae-mediated pulmonary vascular hyperpermeability.Circ Res. 2008 Jun 20;102(12):e120-31. doi: 10.1161/CIRCRESAHA.107.167486. Epub 2008 May 29. Circ Res. 2008. PMID: 18511851 Free PMC article.
-
Role of caveolin-1 in the regulation of pulmonary endothelial permeability.Methods Mol Biol. 2011;763:303-17. doi: 10.1007/978-1-61779-191-8_21. Methods Mol Biol. 2011. PMID: 21874461
-
Rap1 Small GTPase Regulates Vascular Endothelial-Cadherin-Mediated Endothelial Cell-Cell Junctions and Vascular Permeability.Biol Pharm Bull. 2021;44(10):1371-1379. doi: 10.1248/bpb.b21-00504. Biol Pharm Bull. 2021. PMID: 34602545 Review.
-
Dynamic Regulation of Vascular Permeability by Vascular Endothelial Cadherin-Mediated Endothelial Cell-Cell Junctions.J Nippon Med Sch. 2017;84(4):148-159. doi: 10.1272/jnms.84.148. J Nippon Med Sch. 2017. PMID: 28978894 Review.
Cited by
-
SOD2 and the Mitochondrial UPR: Partners Regulating Cellular Phenotypic Transitions.Trends Biochem Sci. 2016 Jul;41(7):568-577. doi: 10.1016/j.tibs.2016.04.004. Epub 2016 May 11. Trends Biochem Sci. 2016. PMID: 27180143 Free PMC article.
-
Depletion of Arg/Abl2 improves endothelial cell adhesion and prevents vascular leak during inflammation.Angiogenesis. 2021 Aug;24(3):677-693. doi: 10.1007/s10456-021-09781-x. Epub 2021 Mar 26. Angiogenesis. 2021. PMID: 33770321 Free PMC article.
-
Endothelial Transcytosis in Acute Lung Injury: Emerging Mechanisms and Therapeutic Approaches.Front Physiol. 2022 Mar 31;13:828093. doi: 10.3389/fphys.2022.828093. eCollection 2022. Front Physiol. 2022. PMID: 35431977 Free PMC article. Review.
-
Cav-1 Protein Levels in Serum and Infarcted Brain Correlate with Hemorrhagic Volume in a Mouse Model of Thromboembolic Stroke, Independently of rt-PA Administration.Mol Neurobiol. 2022 Feb;59(2):1320-1332. doi: 10.1007/s12035-021-02644-y. Epub 2022 Jan 5. Mol Neurobiol. 2022. PMID: 34984586
-
Tyrosine Kinases and Endothelial Homeostasis in Pulmonary Arterial Hypertension: Too Hot to Handle?Am J Respir Cell Mol Biol. 2022 Aug;67(2):147-149. doi: 10.1165/rcmb.2022-0122ED. Am J Respir Cell Mol Biol. 2022. PMID: 35580152 Free PMC article. No abstract available.
References
-
- Lum H, Roebuck KA. Oxidant stress and endothelial cell dysfunction. Am J Physiol. 2001;280:C719–741. - PubMed
-
- Dejana E. Endothelial cell-cell junctions: happy together. Nat Rev Mol Cell Biol. 2004;(5):261–270. - PubMed
-
- Lampugnani MG, Corada M, Caveda L, Breviario F, Ayalon O, Geiger B, Dejana E. The molecular organization of endothelial cell-to-cell junctions: differential association of plakoglobin, β-catenin, and α-catenin with vascular endothelial cadherin (VE-cadherin) J Cell Biol. 1995;129:203–217. - PMC - PubMed
-
- Mehta D, Malik AB. Signaling mechanisms regulating endothelial permeability. Physiol Rev. 2006;86:279–367. - PubMed
-
- Cai H. Hydrogen peroxide regulation of endothelial function: origins, mechanisms, and consequences. Cardiovasc Res. 2005;68:26–36. - PubMed
Publication types
MeSH terms
Substances
Grants and funding
- R01 HL071626-02/HL/NHLBI NIH HHS/United States
- P01 HL060678-069003/HL/NHLBI NIH HHS/United States
- R01 HL071626-03/HL/NHLBI NIH HHS/United States
- P01 HL060678-089003/HL/NHLBI NIH HHS/United States
- P01 HL060678-079003/HL/NHLBI NIH HHS/United States
- P01 HL060678-099003/HL/NHLBI NIH HHS/United States
- R01 HL071626-06/HL/NHLBI NIH HHS/United States
- 5R01 HL071626/HL/NHLBI NIH HHS/United States
- P01 HL060678-109003/HL/NHLBI NIH HHS/United States
- P01 HL060678-100005/HL/NHLBI NIH HHS/United States
- P01 HL060678-090005/HL/NHLBI NIH HHS/United States
- R01 HL071626-01/HL/NHLBI NIH HHS/United States
- R01 HL071626-06S1/HL/NHLBI NIH HHS/United States
- R01 HL071626/HL/NHLBI NIH HHS/United States
- R01 HL071626-05A1/HL/NHLBI NIH HHS/United States
- P01 HL060678-060005/HL/NHLBI NIH HHS/United States
- P01 HL060678-070005/HL/NHLBI NIH HHS/United States
- R01 HL071626-04/HL/NHLBI NIH HHS/United States
- P01 HL060678/HL/NHLBI NIH HHS/United States
- P01 HL060678-080005/HL/NHLBI NIH HHS/United States
LinkOut - more resources
Full Text Sources
Other Literature Sources
Molecular Biology Databases