Ionizing radiation-dependent and independent phosphorylation of the 32-kDa subunit of replication protein A during mitosis
- PMID: 19671522
- PMCID: PMC2764457
- DOI: 10.1093/nar/gkp605
Ionizing radiation-dependent and independent phosphorylation of the 32-kDa subunit of replication protein A during mitosis
Abstract
The human single-stranded DNA-binding protein, replication protein A (RPA), is regulated by the N-terminal phosphorylation of its 32-kDa subunit, RPA2. RPA2 is hyperphosphorylated in response to various DNA-damaging agents and also phosphorylated in a cell-cycle-dependent manner during S- and M-phase, primarily at two CDK consensus sites, S23 and S29. Here we generated two monoclonal phospho-specific antibodies directed against these CDK sites. These phospho-specific RPA2-(P)-S23 and RPA2-(P)-S29 antibodies recognized mitotically phosphorylated RPA2 with high specificity. In addition, the RPA2-(P)-S23 antibody recognized the S-phase-specific phosphorylation of RPA2, suggesting that during S-phase only S23 is phosphorylated, whereas during M-phase both CDK sites, S23 and S29, are phosphorylated. Immunofluorescence microscopy revealed that the mitotic phosphorylation of RPA2 starts at the onset of mitosis, and dephosphorylation occurs during late cytokinesis. In mitotic cells treated with ionizing radiation (IR), we observed a rapid hyperphosphorylation of RPA2 in addition to its mitotic phosphorylation at S23 and S29, associated with a significant change in the subcellular localization of RPA. Our data also indicate that the RPA2 hyperphosphorylation in response to IR is facilitated by the activity of both ATM and DNA-PK, and is associated with activation of the Chk2 pathway.
Figures
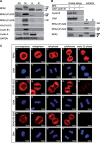
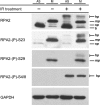
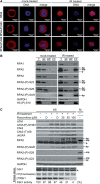
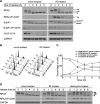
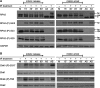
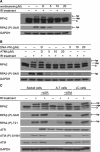
Similar articles
-
Replication protein A2 phosphorylation after DNA damage by the coordinated action of ataxia telangiectasia-mutated and DNA-dependent protein kinase.Cancer Res. 2001 Dec 1;61(23):8554-63. Cancer Res. 2001. PMID: 11731442
-
Differential involvement of phosphatidylinositol 3-kinase-related protein kinases in hyperphosphorylation of replication protein A2 in response to replication-mediated DNA double-strand breaks.Genes Cells. 2006 Mar;11(3):237-46. doi: 10.1111/j.1365-2443.2006.00942.x. Genes Cells. 2006. PMID: 16483312
-
RPA phosphorylation facilitates mitotic exit in response to mitotic DNA damage.Proc Natl Acad Sci U S A. 2008 Sep 2;105(35):12903-8. doi: 10.1073/pnas.0803001105. Epub 2008 Aug 22. Proc Natl Acad Sci U S A. 2008. PMID: 18723675 Free PMC article.
-
Sequential and synergistic modification of human RPA stimulates chromosomal DNA repair.J Biol Chem. 2007 Dec 7;282(49):35910-23. doi: 10.1074/jbc.M704645200. Epub 2007 Oct 10. J Biol Chem. 2007. PMID: 17928296
-
Distinct roles for DNA-PK, ATM and ATR in RPA phosphorylation and checkpoint activation in response to replication stress.Nucleic Acids Res. 2012 Nov;40(21):10780-94. doi: 10.1093/nar/gks849. Epub 2012 Sep 12. Nucleic Acids Res. 2012. PMID: 22977173 Free PMC article.
Cited by
-
Interplay of DNA damage and cell cycle signaling at the level of human replication protein A.DNA Repair (Amst). 2014 Sep;21:12-23. doi: 10.1016/j.dnarep.2014.05.005. Epub 2014 Jun 13. DNA Repair (Amst). 2014. PMID: 25091156 Free PMC article.
-
The Intriguing Mystery of RPA Phosphorylation in DNA Double-Strand Break Repair.Genes (Basel). 2024 Jan 27;15(2):167. doi: 10.3390/genes15020167. Genes (Basel). 2024. PMID: 38397158 Free PMC article. Review.
-
The role of RPA2 phosphorylation in homologous recombination in response to replication arrest.Carcinogenesis. 2010 Jun;31(6):994-1002. doi: 10.1093/carcin/bgq035. Epub 2010 Feb 3. Carcinogenesis. 2010. PMID: 20130019 Free PMC article.
-
DNA damage response is suppressed by the high cyclin-dependent kinase 1 activity in mitotic mammalian cells.J Biol Chem. 2011 Oct 14;286(41):35899-35905. doi: 10.1074/jbc.M111.267690. Epub 2011 Aug 30. J Biol Chem. 2011. PMID: 21878640 Free PMC article.
-
RING finger and WD repeat domain 3 (RFWD3) associates with replication protein A (RPA) and facilitates RPA-mediated DNA damage response.J Biol Chem. 2011 Jun 24;286(25):22314-22. doi: 10.1074/jbc.M111.222802. Epub 2011 May 9. J Biol Chem. 2011. PMID: 21558276 Free PMC article.
References
-
- Sancar A, Lindsey-Boltz LA, Unsal-Kacmaz K, Linn S. Molecular mechanisms of mammalian DNA repair and the DNA damage checkpoints. Annu. Rev. Biochem. 2004;73:39–85. - PubMed
-
- Giaccia AJ, Kastan MB. The complexity of p53 modulation: emerging patterns from divergent signals. Genes Dev. 1998;12:2973–2983. - PubMed
-
- Nasheuer HP, Smith R, Bauerschmidt C, Grosse F, Weisshart K. Initiation of eukaryotic DNA replication: regulation and mechanisms. Prog. Nucleic Acid Res. Mol. Biol. 2002;72:41–94. - PubMed
-
- Sakasai R, Shinohe K, Ichijima Y, Okita N, Shibata A, Asahina K, Teraoka H. Differential involvement of phosphatidylinositol 3-kinase-related protein kinases in hyperphosphorylation of replication protein A2 in response to replication-mediated DNA double-strand breaks. Genes Cells. 2006;11:237–246. - PubMed
-
- Wold MS. Replication protein A: a heterotrimeric, single-stranded DNA-binding protein required for eukaryotic DNA metabolism. Annu. Rev. Biochem. 1997;66:61–92. - PubMed
Publication types
MeSH terms
Substances
LinkOut - more resources
Full Text Sources
Other Literature Sources
Molecular Biology Databases
Research Materials
Miscellaneous