The Fanconi anemia protein FANCM is controlled by FANCD2 and the ATR/ATM pathways
- PMID: 19633289
- PMCID: PMC2757957
- DOI: 10.1074/jbc.M109.007690
The Fanconi anemia protein FANCM is controlled by FANCD2 and the ATR/ATM pathways
Abstract
Genomic stability requires a functional Fanconi anemia (FA) pathway composed of an upstream "core complex" (FA proteins A/B/C/E/F/G/L/M) that mediates monoubiquitination of the downstream targets FANCD2 and FANCI. Unique among FA core complex members, FANCM has processing activities toward replication-associated DNA structures, suggesting a vital role for FANCM during replication. Using Xenopus egg extracts, we analyzed the functions of FANCM in replication and the DNA damage response. xFANCM binds chromatin in a replication-dependent manner and is phosphorylated in response to DNA damage structures. Chromatin binding and DNA damage-induced phosphorylation of xFANCM are mediated in part by the downstream FA pathway protein FANCD2. Moreover, phosphorylation and chromatin recruitment of FANCM is regulated by two mayor players in the DNA damage response: the cell cycle checkpoint kinases ATR and ATM. Our results indicate that functions of FANCM are controlled by FA- and non-FA pathways in the DNA damage response.
Figures
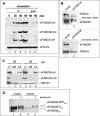
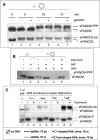
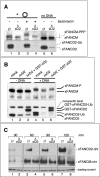
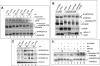
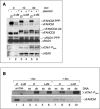
Similar articles
-
Fanconi anemia proteins FANCD2 and FANCI exhibit different DNA damage responses during S-phase.Nucleic Acids Res. 2012 Sep 1;40(17):8425-39. doi: 10.1093/nar/gks638. Epub 2012 Jun 29. Nucleic Acids Res. 2012. PMID: 22753026 Free PMC article.
-
Fanconi anemia proteins are required to prevent accumulation of replication-associated DNA double-strand breaks.Mol Cell Biol. 2006 Jan;26(2):425-37. doi: 10.1128/MCB.26.2.425-437.2006. Mol Cell Biol. 2006. PMID: 16382135 Free PMC article.
-
The FANCM/FAAP24 complex is required for the DNA interstrand crosslink-induced checkpoint response.Mol Cell. 2010 Jul 30;39(2):259-68. doi: 10.1016/j.molcel.2010.07.005. Mol Cell. 2010. PMID: 20670894 Free PMC article.
-
Regulation of the Fanconi Anemia DNA Repair Pathway by Phosphorylation and Monoubiquitination.Genes (Basel). 2021 Nov 5;12(11):1763. doi: 10.3390/genes12111763. Genes (Basel). 2021. PMID: 34828369 Free PMC article. Review.
-
The Fanconi anemia ID2 complex: dueling saxes at the crossroads.Cell Cycle. 2014;13(19):2999-3015. doi: 10.4161/15384101.2014.956475. Cell Cycle. 2014. PMID: 25486561 Free PMC article. Review.
Cited by
-
DNA interstrand cross-link repair requires replication-fork convergence.Nat Struct Mol Biol. 2015 Mar;22(3):242-7. doi: 10.1038/nsmb.2956. Epub 2015 Feb 2. Nat Struct Mol Biol. 2015. PMID: 25643322 Free PMC article.
-
Cellular response to DNA interstrand crosslinks: the Fanconi anemia pathway.Cell Mol Life Sci. 2016 Aug;73(16):3097-114. doi: 10.1007/s00018-016-2218-x. Epub 2016 Apr 19. Cell Mol Life Sci. 2016. PMID: 27094386 Free PMC article. Review.
-
DNA damage response: three levels of DNA repair regulation.Cold Spring Harb Perspect Biol. 2013 Aug 1;5(8):a012724. doi: 10.1101/cshperspect.a012724. Cold Spring Harb Perspect Biol. 2013. PMID: 23813586 Free PMC article. Review.
-
Bloom syndrome complex promotes FANCM recruitment to stalled replication forks and facilitates both repair and traverse of DNA interstrand crosslinks.Cell Discov. 2016 Dec 20;2:16047. doi: 10.1038/celldisc.2016.47. eCollection 2016. Cell Discov. 2016. PMID: 28058110 Free PMC article.
-
Monoketone analogs of curcumin, a new class of Fanconi anemia pathway inhibitors.Mol Cancer. 2009 Dec 31;8:133. doi: 10.1186/1476-4598-8-133. Mol Cancer. 2009. PMID: 20043851 Free PMC article.
References
Publication types
MeSH terms
Substances
Grants and funding
LinkOut - more resources
Full Text Sources
Research Materials
Miscellaneous