Expression of RNA CCUG repeats dysregulates translation and degradation of proteins in myotonic dystrophy 2 patients
- PMID: 19590039
- PMCID: PMC2716970
- DOI: 10.2353/ajpath.2009.090047
Expression of RNA CCUG repeats dysregulates translation and degradation of proteins in myotonic dystrophy 2 patients
Abstract
Myotonic dystrophy 2 (DM2) is a multisystem skeletal muscle disease caused by an expansion of tetranucleotide CCTG repeats, the transcription of which results in the accumulation of untranslated CCUG RNA. In this study, we report that CCUG repeats both bind to and misregulate the biological functions of cytoplasmic multiprotein complexes. Two CCUG-interacting complexes were subsequently purified and analyzed. A major component of one of the complexes was found to be the 20S catalytic core complex of the proteasome. The second complex was found to contain CUG triplet repeat RNA-binding protein 1 (CUGBP1) and the translation initiation factor eIF2. Consistent with the biological functions of the 20S proteasome and the CUGBP1-eIF2 complexes, the stability of short-lived proteins and the levels of the translational targets of CUGBP1 were shown to be elevated in DM2 myoblasts. We found that the overexpression of CCUG repeats in human myoblasts from unaffected patients, in C2C12 myoblasts, and in a DM2 mouse model alters protein translation and degradation, similar to the alterations observed in DM2 patients. Taken together, these findings show that RNA CCUG repeats misregulate protein turnover on both the levels of translation and proteasome-mediated protein degradation.
Figures
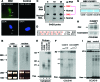
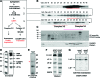
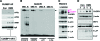
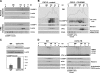
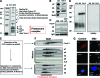
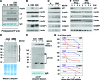
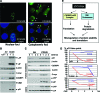
Similar articles
-
RNA Foci, CUGBP1, and ZNF9 are the primary targets of the mutant CUG and CCUG repeats expanded in myotonic dystrophies type 1 and type 2.Am J Pathol. 2011 Nov;179(5):2475-89. doi: 10.1016/j.ajpath.2011.07.013. Epub 2011 Sep 1. Am J Pathol. 2011. PMID: 21889481 Free PMC article.
-
CCUG repeats reduce the rate of global protein synthesis in myotonic dystrophy type 2.Rev Neurosci. 2010;21(1):19-28. doi: 10.1515/revneuro.2010.21.1.19. Rev Neurosci. 2010. PMID: 20458885 Review.
-
(CCUG)n RNA toxicity in a Drosophila model of myotonic dystrophy type 2 (DM2) activates apoptosis.Dis Model Mech. 2017 Aug 1;10(8):993-1003. doi: 10.1242/dmm.026179. Epub 2017 Jun 16. Dis Model Mech. 2017. PMID: 28623239 Free PMC article.
-
Reduction of the rate of protein translation in patients with myotonic dystrophy 2.J Neurosci. 2009 Jul 15;29(28):9042-9. doi: 10.1523/JNEUROSCI.1983-09.2009. J Neurosci. 2009. PMID: 19605641 Free PMC article.
-
Dysfunction of protein homeostasis in myotonic dystrophies.Histol Histopathol. 2013 Sep;28(9):1089-98. doi: 10.14670/HH-28.1089. Epub 2013 Mar 28. Histol Histopathol. 2013. PMID: 23536431 Review.
Cited by
-
Differences in aberrant expression and splicing of sarcomeric proteins in the myotonic dystrophies DM1 and DM2.Acta Neuropathol. 2010 Apr;119(4):465-79. doi: 10.1007/s00401-010-0637-6. Epub 2010 Jan 12. Acta Neuropathol. 2010. PMID: 20066428 Free PMC article.
-
RNA Foci, CUGBP1, and ZNF9 are the primary targets of the mutant CUG and CCUG repeats expanded in myotonic dystrophies type 1 and type 2.Am J Pathol. 2011 Nov;179(5):2475-89. doi: 10.1016/j.ajpath.2011.07.013. Epub 2011 Sep 1. Am J Pathol. 2011. PMID: 21889481 Free PMC article.
-
Myotonic dystrophy type 2 and modifier genes: an update on clinical and pathomolecular aspects.Neurol Sci. 2017 Apr;38(4):535-546. doi: 10.1007/s10072-016-2805-5. Epub 2017 Jan 11. Neurol Sci. 2017. PMID: 28078562 Review.
-
RNA toxicity in non-coding repeat expansion disorders.EMBO J. 2020 Jan 2;39(1):e101112. doi: 10.15252/embj.2018101112. Epub 2019 Nov 13. EMBO J. 2020. PMID: 31721251 Free PMC article. Review.
-
The origin of genetic instability in CCTG repeats.Nucleic Acids Res. 2011 Aug;39(14):6260-8. doi: 10.1093/nar/gkr185. Epub 2011 Apr 7. Nucleic Acids Res. 2011. PMID: 21478167 Free PMC article.
References
-
- Harper PS. Myotonic dystrophy. London: W.B. Saunders,; 2001
-
- Aslanidis C, Jansen G, Amemiya C, Shutler G, Mahadevan M, Tsilfidis C, Clen C, Alleman J, Wormskamp NG, Vooijs M, Buxton J, Johnson K, Sweets HLM, Lennon GG, Carrano AV, Korneluk RG, Wieringa B, deJong PJ. Cloning of essential myotonic dystrophy region and mapping of the putative defect. Nature. 1992;355:548–551. - PubMed
-
- Liquori CL, Ricker K, Moseley ML, Jacobsen JF, Kress W, Naylor SL, Day JW, Ranum L. Myotonic dystrophy type 2 caused by a CCTG expansion in intron of ZNF9. Science. 2001;293:864–867. - PubMed
-
- Day JW, Ricker K, Jacobsen JF, Rasmussen LJ, Dick KA, Kress W, Schneider C, Koch MC, Beilman GJ, Harrison , Dalton JC, Ranum LP. Myotonic dystrophy type 2: molecular, diagnostic and clinical spectrum. Neurol. 2003;60:657–664. - PubMed
Publication types
MeSH terms
Substances
Grants and funding
LinkOut - more resources
Full Text Sources
Research Materials
Miscellaneous